Identifiable effects on public health which may be expected from the presence of a pollutant in ambient air, e.g. Heart Attacks
Submitted by Norm Roulet on Mon, 06/07/2010 - 11:00.
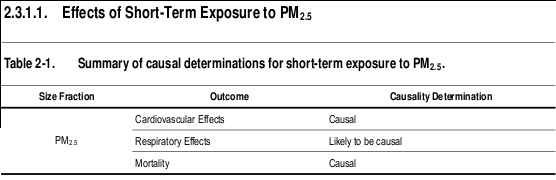
As a result of old science, politics and industry dominating energy, health and environmental planning and development of Cleveland, Northeast Ohio, Ohio and America, citizens here must confront the realities of too much pollution in our air today, with certainty of growing air pollution worldwide in the years ahead. As such, the United States Environmental Protection Agency's 2009 Integrated Science Assessment for Particulate Matter finds our pollution causes cardiovascular and respiratory problems and death... topping a long list of cumulative harm pollution causes people and society. Integrated Science Assessment for Particulate Matter forms the scientific foundation for the review of the primary (health-based) and secondary (welfare-based) National Ambient Air Quality Standards (NAAQS) for particulate matter (PM) in America, and "accurately reflects “the latest scientific knowledge useful in indicating the kind and extent of identifiable effects on public health which may be expected from the presence of [a] pollutant in ambient air”".
As I've long written on realNEO, Northeast Ohio has a pollution crisis and does a poor job or monitoring our pollution, putting citizens' lives in danger. How much in danger is the subject of this lengthy EPA analysis. In short, you are certainly being harmed greatly by the high levels of PM clearly released into the air in Northeast Ohio, especially near major roadways and coal burning facilities that are source points, like Mittal and MCCO. For example: "Epidemiologic studies that examined the effect of PM 2.5 on cardiovascular emergency department (ED) visits and hospital admissions reported consistent positive associations (predominantly for ischemic heart disease [IHD] and congestive heart failure [CHF]), with the majority of studies reporting increases ranging from 0.5 to 3.4% per 10 μg/m3 increase in PM 2.5".
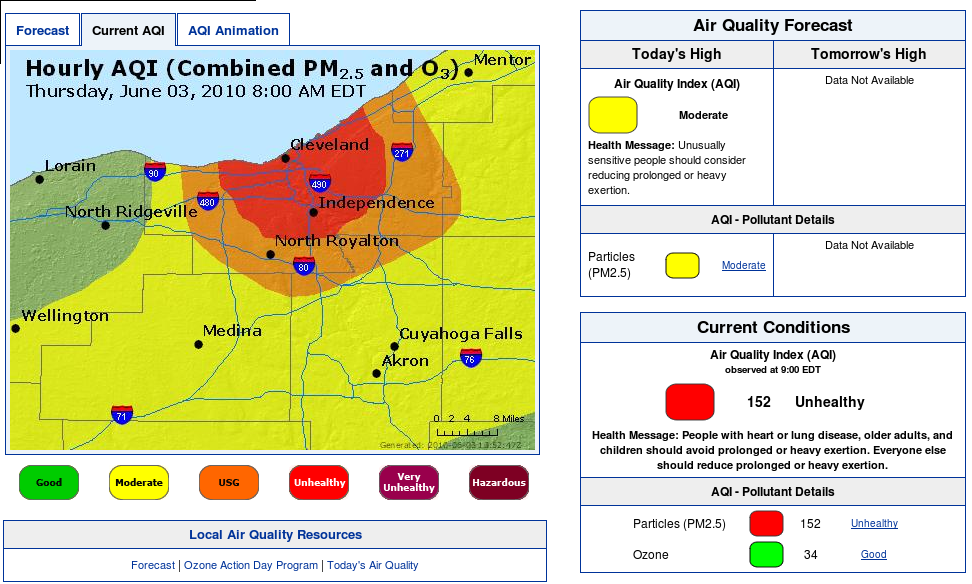
On Memorial Day, 2010, pollution monitors in the Tremont area of Cleveland reported PM 2.5 readings in excess of 317 μg/m3 - nearly 6 times the EPA ambient standard 24 hour concentration of 65 ug per cubic meter Primary and Secondary... more than 21X the annual mean standard of 15 ug per cubic meter... does that mean sleeping residents were 15-90+% more likely to have episodes requiring cardiovascular emergency department (ED) visits and hospital admissions, and within what time frame?
If that reality isn't front and center in your brain, perhaps the pollution has taken control of your mind... you need to read this report - note huge file sizes: Integrated Science Assessment for Particulate Matter (Full Report) (2228 pp, 105.4 MB) - Integrated Science Assessment for Particulate Matter (Final Report Without Annexes) (PDF) (1071 pp, 48 MB).
I've summarized and included below some important highlights of this 2000+ page report... like findings related to Table 2-1 above - Summary of causal determinations for short-term exposure to PM 2.5 - concluding, among many causal things, "the collective evidence from epidemiologic, controlled human exposure, and toxicological studies is sufficient to conclude that a causal relationship exists between short-term exposures to PM 2.5 and cardiovascular effects." In other words, our air pollution is as serious as a heart attack.
One especially relevant finding I mined from page 119:
Spatial variability in source contributions across urban areas is an important consideration in assessing the likelihood of exposure error in epidemiologic studies relating health outcomes to sources. Concepts similar to those for using ambient concentrations as surrogates for personal exposures apply here. Some source attribution studies for PM 2.5 indicate that intra-urban variability increases in the following order: regional sources (e.g., secondary SO 4 2– originating from EGUs) < area sources (e.g., on-road mobile sources) < point sources (e.g., metals from stacks of smelters).
Although limited information was available for PM 10-2.5 , it does indicate a similar ordering, but without a regional component (resulting from the short lifetime of PM 10-2.5 compared to transport times on the regional scale). More discussion on source contributions to PM is available in Section 3.6.
That makes living near point sources (e.g., metals from stacks of smelters) especially significant. Anyone in Cleveland live near major PM 2.5 point sources? Here are some other highlights to think about:
"The degree of spatial variability in PM was likely to be region-specific and strongly influenced by local sources and meteorological and topographic conditions (p116)." "In general, PM 2.5 has a longer atmospheric lifetime than PM 10-2.5 . As a result, PM 2.5 is more homogeneously distributed than PM 10-2.5 , whose concentrations more closely reflect proximity to local sources (Section 3.5.1.2)". "UFPs are not measured as part of AQS or any other routine regulatory network in the U.S. Therefore, information about the spatial variability of UFPs is sparse; however, their number concentrations are expected to be highly spatially and temporally variable. This has been shown on the urban scale in studies in which UFP number concentrations drop off quickly with distance from roads compared to accumulation mode particle numbers."
"Correlations between PM and gaseous copollutants, including SO 2 , NO 2 , carbon monoxide (CO) and O 3 , varied both seasonally and spatially between and within metropolitan areas (Section 3.5.3)." "The correlation between daily maximum 8-h avg O 3 and 24-h avg PM 2.5 showed the highest degree of seasonal variability with positive correlations on average in summer (avg = 0.56) and negative correlations on average in the winter (avg = -0.30). During the transition seasons, spring and fall, correlations were mixed but on average were still positive. PM 2.5 is both primary and secondary in origin, whereas O 3 is only secondary. Photochemical production of O 3 and secondary PM in the planetary boundary layer (PBL) is much slower during the winter than during other seasons. Primary pollutant concentrations (e.g., primary PM 2.5 components, NO and NO 2 ) in many urban areas are elevated in winter as the result of heating emissions, cold starts and low mixing heights. O 3 in the PBL during winter is mainly associated with air subsiding from above the boundary layer following the passage of cold fronts, and this subsiding air has much lower PM concentrations than are present in the PBL. Therefore, a negative association between O 3 and PM 2.5 is frequently observed in the winter. During summer, both O 3 and secondary PM 2.5 are produced in the PBL and in the lower free troposphere at faster rates compared to winter, and so they tend to be positively correlated."
"The federal reference methods (FRMs) for PM 2.5 and PM 10 are based on criteria outlined in the Code of Federal Regulations. They are, however, subject to several limitations that should be kept in mind when using compliance monitoring data for health studies. For example, FRM techniques are subject to the loss of semi-volatile species such as organic compounds and ammonium nitrate (especially in the West). Since FRMs based on gravimetry use 24-h integrated filter samples to collect PM mass, no information is available for variations over shorter averaging times from these instruments. However, methods have been developed to measure real-time PM mass concentrations. Real-time (or continuous and semi-continuous) measurement techniques are also available for PM species, such as particle into liquid sampler (PILS) for multiple ions analysis and aerosol mass spectrometer (AMS) for multiple components analysis (Section 3.4.1). Advances have also been achieved in PM organic speciation. New 24-h FRMs and Federal Equivalent Methods (FEMs) based on gravimetry and continuous FEMs for PM 10-2.5 are available. FRMs for PM 10-2.5 rely on calculating the difference between co-located PM 10 and PM 2.5 measurements while a dichotomous sampler is designated as an FEM."
"Results of receptor modeling calculations indicate that PM 2.5 is produced mainly by combustion of fossil fuel, either by stationary sources or by transportation. A relatively small number of broadly defined source categories, compared to the total number of chemical species that typically are measured in ambient monitoring source receptor studies, account for the majority of the observed PM mass. Some ambiguity is inherent in identifying source categories. For example, quite different mobile sources such as trucks, farm equipment, and locomotives rely on diesel engines and ancillary data is often required to resolve these sources. A compilation of study results shows that secondary SO 4 2– (derived mainly from SO 2 emitted by Electricity Generating Units [EGUs]), NO 3 – (from the oxidation of NO x emitted mainly from transportation sources and EGUs), and primary mobile source categories, constitute most of PM 2.5 (and PM 10 ) in the East. PM 10-2.5 is mainly primary in origin, having been emitted as fully formed particles derived from abrasion and crushing processes, soil disturbances, plant and insect fragments, pollens and other microorganisms, desiccation of marine aerosol emitted from bursting bubbles, and hygroscopic fine PM expanding with humidity to coarse mode. Gases such as HNO 3 can also condense directly onto preexisting coarse particles. Suspended primary coarse PM can contain Fe, Si, Al, and base cations from soil, plant and insect fragments, pollen, fungal spores, bacteria, and viruses, as well as fly ash, brake lining particles, debris, and automobile tire fragments. Quoted uncertainties in the source apportionment of constituents in ambient aerosol samples typically range from 10 to 50%. An intercomparison of source apportionment techniques indicated that the same major source categories of PM 2.5 were consistently identified by several independent groups working with the same data sets. Soil-, sulfate-, residual oil-, and salt-associated mass were most clearly identified by the groups. Other sources with more ambiguous signatures, such as vegetative burning and traffic-related emissions were less consistently identified."
"Spatial variability in source contributions across urban areas is an important consideration in assessing the likelihood of exposure error in epidemiologic studies relating health outcomes to sources. Concepts similar to those for using ambient concentrations as surrogates for personal exposures apply here. Some source attribution studies for PM 2.5 indicate that intra-urban variability increases in the following order: regional sources (e.g., secondary SO 4 2– originating from EGUs) < area sources (e.g., on-road mobile sources) < point sources (e.g., metals from stacks of smelters). Although limited information was available for PM 10-2.5 , it does indicate a similar ordering, but without a regional component (resulting from the short lifetime of PM 10-2.5 compared to transport times on the regional scale). More discussion on source contributions to PM is available in Section 3.6."
As I have previously reported on realNEO, "Short-Term Exposure To Fine Particle Air Pollution Can Drive Up High Blood Pressure, Raise Risk Of Heart Attack", "A Healthy Brain Is Essential For Successful, Healthy Aging: Air Pollution Contributes To The Risk Of Alzheimer’s-Type Disease" and, in "Cover letter to President Obama from the 2008–2009 Annual Report of the President’s Cancer Panel, April 2010", surface: "In 2009 alone, approximately 1.5 million American men, women, and children were diagnosed with cancer, and 562,000 died from the disease. With the growing body of evidence linking environmental exposures to cancer, the public is becoming increasingly aware of the unacceptable burden of cancer resulting from environmental and occupational exposures that could have been prevented through appropriate national action."
In summary conclusion, Integrated Science Assessment for Particulate Matter finds, suspects and is investigating causal relationships between our air pollution and all the following concerns for our community:
2.6. Summary of Health Effects and Welfare Effects
Causal Determinations
This chapter has provided an overview of the underlying evidence used in making the causal determinations for the health and welfare effects and PM size fractions evaluated. This review builds upon the main conclusions of the last PM AQCD (U.S. EPA, 2004, 056905):
- "A growing body of evidence both from epidemiological and toxicological studies supports the general conclusion that PM 2.5 (or one or more PM 2.5 components), acting alone and/or in
- “A much more limited body of evidence is suggestive of associations between short-term (but not long-term) exposures to ambient coarse-fraction thoracic particles and various mortality and morbidity effects observed at times in some locations. This suggests that PM 10-2.5 , or some constituent component(s) of PM 10-2.5 , may contribute under some circumstances to increased human health risks with somewhat stronger evidence for associations with morbidity (especially respiratory) endpoints than for mortality.” (pg 9-79 and 9-80)
- "Impairment of visibility in rural and urban areas is directly related to ambient concentrations of fine particles, as modulated by particle composition, size, and hygroscopic characteristics, and by relative humidity.” (pg 9-99)
- “Available evidence, ranging from satellite to in situ measurements of aerosol effects on incoming solar radiation and cloud properties, is strongly indicative of an important role in climate for aerosols, but this role is still poorly quantified.” (pg 9-111)
The evaluation of the epidemiologic, toxicological, and controlled human exposure studies published since the completion of the 2004 PM AQCD have provided additional evidence for PM-related health effects. Table 2-6 provides an overview of the causal determinations for all PM size fractions and health effects. Causal determinations for PM and welfare effects, including visibility, climate, ecological effects, and materials are included in Table 2-7. Detailed discussions of the scientific evidence and rationale for these causal determinations are provided in the subsequent chapters of this ISA.

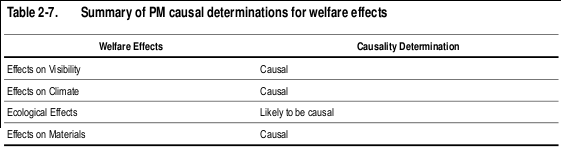
As explained in its introduction, the focus of this 2228 page Integrated Science Assessment for Particulate Matter is on scientific evidence that is most relevant to the following questions that have been taken directly from the Integrated Review Plan:
- Has new information altered the body of scientific support for the occurrence of health effects following short- and/or long-term exposure to levels of fine and thoracic coarse particles found in the ambient air?
- Has new information altered conclusions from previous reviews regarding the plausibility of adverse health effects associated with exposures to PM 2.5 , PM 10 , PM 10-2.5 , or alternative PM indicators that might be considered?
- What evidence is available from recent studies focused on specific size fractions, chemical components, sources, or environments (e.g., urban and non-urban areas) of PM to inform our understanding of the nature of PM exposures that are linked to various health outcomes?
- To what extent is key scientific evidence becoming available to improve our understanding of the health effects associated with various time periods of PM exposures, including not only short-term (daily or multi-day) and chronic (months to years) exposures, but also peak PM exposures (<24 hours)? To what extent is critical research becoming available that could improve our understanding of the relationship between various health endpoints and different lag periods (e.g., <1 day, single day, multi-day distributed lags)?
- What data are available to improve our understanding of spatial and/or temporal heterogeneity of PM exposures considering different size fractions and/or components?
- At what levels of PM exposure do health effects of concern occur? Is there evidence for the occurrence of adverse health effects at levels of PM lower than those observed previously? If so, at what levels and what are the important uncertainties associated with that evidence? What is the nature of the dose-response relationships of PM for the various health effects evaluated?
- What evidence is available linking particle number concentration with adverse health effects of UF particles?
- Do risk/exposure estimates suggest that exposures of concern for PM-induced health effects will occur with current ambient levels of PM or with levels that just meet the current standards? If so, are these risks/exposures of sufficient magnitude such that the health effects might reasonably be judged to be important from a public health perspective? What are the important uncertainties associated with these risk/exposure estimates?
- To what extent is key evidence becoming available that could inform our understanding of subpopulations that are particularly sensitive or vulnerable to PM exposures? In the last review, sensitive or vulnerable subpopulations that appeared to be at greater risk for
- PM-related effects included individuals with pre-existing heart and lung diseases, older adults, and children. Has new evidence become available to suggest additional sensitive subpopulations should be given increased focus in this review (e.g., fetuses, neonates, genetically susceptible subpopulations)?
- To what extent is key evidence becoming available to inform our understanding of populations that are particularly vulnerable to PM exposures? Specifically, is there new or emerging evidence to inform our understanding of geographical, spatial, SES, and environmental justice considerations?
- To what extent have important uncertainties identified in the last review been reduced and/or have new uncertainties emerged?
- To what extent is new information available to inform our understanding of non-PM- exposure factors that might influence the associations between PM levels and health effects being considered (e.g., weather-related factors; behavioral factors such as heating/air conditioning use; driving patterns; and time-activity patterns)?
The Integrated Review Plan for the National Ambient Air Quality Standards for Particulate Matter identifies a series of policy-relevant questions that provide a framework for this assessment of the scientific evidence (U.S. EPA, 2008, 157072). These questions frame the entire review of the NAAQS for PM, and thus are informed by both science and policy considerations. The ISA organizes and presents the scientific evidence such that, when considered along with findings from risk analyses and policy considerations, will help the EPA address these questions during the NAAQS review for PM. In evaluating
In evaluating evidence on welfare effects of PM, the focus will be on evidence that can help inform these questions from the Integrated Review Plan:
- What new evidence is available on the relationship between PM mass/size fraction and/or specific PM components and visibility impairment and climate-related and other welfare effects?
- To what extent has key scientific evidence now become available to improve our understanding of the nature and magnitude of visibility, climate, and ecosystem responses to PM and the variability associated with those responses (including ecosystem type, climatic conditions, environmental effects and interactions with other environmental factors and pollutants)?
- Do the evidence, the air quality assessment, and the risk/exposure assessment provide support for considering alternative averaging times?
- At what levels of ambient PM do visibility impairment and/or environmental effects of concern occur? Is there evidence for the occurrence of adverse visibility and other welfare-related effects at levels of PM lower than those observed previously? If so, at what levels and what are the important uncertainties associated with the evidence?
- Do the analyses suggest that PM-induced visibility impairment and/or other welfare- effects will occur with current ambient levels of PM or with levels that just meet the current standards? If so, are these effects of sufficient magnitude and/or frequency such that these effects might reasonably be judged to be important from a public welfare perspective? What are the uncertainties associated with these estimates?
- What new evidence and/or techniques are available to quantify the benefits of improved visibility and/or other welfare-related effects?
- To what extent have important uncertainties identified in the last review been reduced and/or have new uncertainties emerged?
Included below is all of the second chapter of Integrated Science Assessment for Particulate Matter - even if you aren't up to reading through the entire report, skimming through just this overview may change your life forever... I've put some key points in bold:
Integrated Science Assessment for Particulate Matter (Final Report)
Notice
EPA is announcing the availability of the final Integrated Science Assessment for Particulate Matter as described in the December 15, 2009 Federal Register Notice.
EPA has released the final Integrated Science Assessment (ISA) for Particulate Matter (PM). This is EPA’s latest evaluation of the scientific literature on the potential human health and welfare effects associated with ambient exposures to particulate matter (PM). The development of this document is part of the Agency's periodic review of the national ambient air quality standards (NAAQS) for PM. The recently completed PM ISA and supplementary annexes, in conjunction with additional technical and policy assessments developed by EPA’s Office of Air and Radiation, will provide the scientific basis to inform EPA decisions related to the review of the current PM NAAQS.
 PM is one of six principal (or criteria) pollutants for which EPA has established NAAQS. Periodically, EPA reviews the scientific basis for these standards by preparing an ISA (formerly called an Air Quality Criteria Document). The ISA and supplementary annexes, in conjunction with additional technical and policy assessments, provide the scientific basis for EPA decisions on the adequacy of the current NAAQS and the appropriateness of possible alternative standards. The Clean Air Scientific Advisory Committee (CASAC), an independent science advisory committee whose existence and whose review and advisory functions are mandated by Section 109 (d) (2) of the Clean Air Act, is charged (among other things) with independent scientific review of EPA's air quality criteria.
The first and second drafts of the PM ISA were released on December 22, 2008 and July 31, 2009, respectively, for independent external peer review and public comment. These drafts were reviewed at public meetings of the CASAC PM Review Panel on April 1-2, 2009 and October 5-6, 2009, respectively. This final PM ISA has benefited from the expert comments received at the CASAC meetings and from public comments, and it has been revised accordingly.
Next Steps
This is the final document. Additional technical and policy assessment documents for this review are available from the Office of Air Quality Planning and Standards ( http://www.epa.gov/ttn/naaqs/standards/pm/s_pm_index.html).
Contact
- stanek [dot] lindsay [at] epa [dot] gov
- by phone at: 919-541-7792
- by fax at: 919-541-2985
- by email at: stanek [dot] lindsay [at] epa [dot] gov
Background
Chapter 2. Integrative Health and Welfare Effects Overview
The subsequent chapters of this ISA will present the most policy-relevant information related to this review of the NAAQS for PM. This chapter integrates the key findings from the disciplines evaluated in this current assessment of the PM scientific literature, which includes the atmospheric sciences, ambient air data analyses, exposure assessment, dosimetry, health studies (e.g., toxicological, controlled human exposure, and epidemiologic), and welfare effects. The EPA framework for causal determinations described in Chapter 1 has been applied to the body of scientific evidence in order to collectively examine the health or welfare effects attributed to PM exposure in a two-step process.
As described in Chapter 1, EPA assesses the results of recent relevant publications, building upon evidence available during the previous NAAQS reviews, to draw conclusions on the causal relationships between relevant pollutant exposures and health or environmental effects. This ISA uses a five-level hierarchy that classifies the weight of evidence for causation:
- Causal relationship
- Likely to be a causal relationship
- Suggestive of a causal relationship
- Inadequate to infer a causal relationship
- Not likely to be a causal relationship
Beyond judgments regarding causality are questions relevant to quantifying health or environmental risks based on our understanding of the quantitative relationships between pollutant exposures and health or welfare effects. Once a determination is made regarding the causal relationship between the pollutant and outcome category, important questions regarding quantitative relationships include: What is the concentration-response or dose-response relationship? Under what exposure conditions (amount deposited, dose or concentration, duration and pattern) are effects observed? What populations appear to be differentially affected (i.e., more susceptible) to effects? What elements of the ecosystem (e.g., types, regions, taxonomic groups, populations, functions, etc.) appear to be affected, or are more sensitive to effects?
To address these questions, in the second step of the EPA framework, the entirety of quantitative evidence is evaluated to identify and characterize potential concentration-response relationships. This requires evaluation of levels of pollutant and exposure durations at which effects were observed for exposed populations including potentially susceptible populations.
This chapter summarizes and integrates the newly available scientific evidence that best informs consideration of the policy-relevant questions that frame this assessment, presented in Chapter 1. Section 2.1 discusses the trends in ambient concentrations and sources of PM and provides a brief summary of ambient air quality. Section 2.2 presents the evidence regarding personal exposure to ambient PM in outdoor and indoor microenvironments, and it discusses the relationship between ambient PM concentrations and exposure to PM from ambient sources. Section 2.3 integrates the evidence for studies that examine the health effects associated with short-and long-term exposure to PM and discusses important uncertainties identified in the interpretation of the scientific evidence. Section 2.4 provides a discussion of policy-relevant considerations, such as potentially susceptible populations, lag structure, and the PM concentration-response relationship, and PM sources and constituents linked to health effects. Section 2.5 summarizes the evidence for welfare effects related to PM exposure. Finally, Section 2.6 provides all of the causal determinations reached for each of the health outcomes and PM exposure durations evaluated in this ISA.
2.1. Concentrations and Sources of Atmospheric PM 2.1.1. Ambient PM Variability and Correlations
Recently, advances in understanding the spatiotemporal distribution of PM mass and its constituents have been made, particularly with regard to PM 2.5 and its components as well as ultrafine particles (UFPs). Emphasis in this ISA is placed on the period from 2005-2007, incorporating the most recent validated EPA Air Quality System (AQS) data. The AQS is EPA’s repository for ambient monitoring data reported by the national, and state and local air monitoring networks. Measurements of PM 2.5 and PM 10 are reported into AQS, while PM 10-2.5 concentrations are obtained as the difference between PM 10 and PM 2.5 (after converting PM 10 concentrations from STP to local conditions; Section 3.5). Note, however, that a majority of U.S. counties were not represented in AQS because their population fell below the regulatory monitoring threshold. Moreover, monitors reporting to AQS were not uniformly distributed across the U.S. or within counties, and conclusions drawn from AQS data may not apply equally to all parts of a geographic region. Furthermore, biases can exist for some PM constituents (and hence total mass) owing to volatilization losses of nitrates and other semi-volatile compounds, and, conversely, to retention of particle-bound water by hygroscopic species. The degree of spatial variability in PM was likely to be region-specific and strongly influenced by local sources and meteorological and topographic conditions.
2.1.1.1. Spatial Variability across the U.S.
AQS data for daily average concentrations of PM 2.5 for 2005-2007 showed considerable variability across the U.S. (Section 3.5.1.1). Counties with the highest average concentrations of PM 2.5 (>18 μg/m3) were reported for several counties in the San Joaquin Valley and inland southern California as well as Jefferson County, AL (containing Birmingham) and Allegheny County, PA (containing Pittsburgh). Relatively few regulatory monitoring sites have the appropriate co-located monitors for computing PM 10-2.5 , resulting in poor geographic coverage on a national scale (Figure 3-10). Although the general understanding of PM differential settling leads to an expectation of greater spatial heterogeneity in the PM 10-2.5 fraction, deposition of particles as a function of size depends strongly on local meteorological conditions. Better geographic coverage is available for PM 10 , where the highest reported annual average concentrations (>50 μg/m3) occurred in southern California, southern Arizona and central New Mexico. The size distribution of PM varied substantially by location, with a generally larger fraction of PM 10 mass in the PM 10-2.5 size range in western cities (e.g., Phoenix and Denver) and a larger fraction of PM 10 in the PM 2.5 size range in eastern U.S. cities (e.g., Pittsburgh and Philadelphia). UFPs are not measured as part of AQS or any other routine regulatory network in the U.S. Therefore, limited information is available regarding regional variability in the spatiotemporal distribution of UFPs.
Spatial variability in PM 2.5 components obtained from the Chemical Speciation Network (CSN) varied considerably by species from 2005-2007 (Figures 3-12 through 3-18). The highest annual average organic carbon (OC) concentrations were observed in the western and southeastern U.S. OC concentrations in the western U.S. peaked in the fall and winter, while OC concentrations in the Southeast peaked anytime between spring and fall. Elemental carbon (EC) exhibited less seasonality than OC and showed lowest seasonal variability in the eastern half of the U.S. The December 2009 highest annual average EC concentrations were present in Los Angeles, Pittsburgh, New York, and El Paso. Concentrations of sulfate (SO 4 2–) were higher in the eastern U.S. as a result of higher SO 2 emissions in the East compared with the West. There is also considerable seasonal variability with higher SO 4 2– concentrations in the summer months when the oxidation of SO 2 proceeds at a faster rate than during the winter. Nitrate (NO 3 –) concentrations were highest in California and during the winter in the Upper Midwest. In general, NO 3 – was higher in the winter across the country, in part as a result of temperature-driven partitioning and volatilization. Exceptions existed in Los Angeles and Riverside, CA, where high NO 3 – concentrations appeared year-round. There is variation in both PM 2.5 mass and composition among cities, some of which might be due to regional differences in meteorology, sources, and topography.
2.1.1.2. Spatial Variability on the Urban and Neighborhood Scales
In general, PM 2.5 has a longer atmospheric lifetime than PM 10-2.5 . As a result, PM 2.5 is more homogeneously distributed than PM 10-2.5 , whose concentrations more closely reflect proximity to local sources (Section 3.5.1.2). Because PM 10 encompasses PM 10-2.5 in addition to PM 2.5 , it also exhibits more spatial heterogeneity than PM 2.5 . Urban- and neighborhood-scale variability in PM mass and composition was examined by focusing on 15 metropolitan areas, which were chosen based on their geographic distribution and coverage in recent health effects studies. The urban areas selected were Atlanta, Birmingham, Boston, Chicago, Denver, Detroit, Houston, Los Angeles, New York, Philadelphia, Phoenix, Pittsburgh, Riverside, Seattle and St. Louis. Inter-monitor correlation remained higher over long distances for PM 2.5 as compared with PM 10 in these 15 urban areas. To a large extent, greater variation in PM 2.5 and PM 10 concentrations within cities was observed in areas with lower ratios of PM 2.5 to PM 10 . When the data was limited to only sampler pairs with less than 4 km separation (i.e., on a neighborhood scale), inter-sampler correlations remained higher for PM 2.5 than for PM 10 . The average inter-sampler correlation was 0.93 for PM 2.5 , while it dropped to 0.70 for PM 10 (Section 3.5.1.3). Insufficient data were available in the 15 metropolitan areas to perform similar analyses for PM 10-2.5 using co-located, low volume FRM monitors.
As previously mentioned, UFPs are not measured as part of AQS or any other routine regulatory network in the U.S. Therefore, information about the spatial variability of UFPs is sparse; however, their number concentrations are expected to be highly spatially and temporally variable. This has been shown on the urban scale in studies in which UFP number concentrations drop off quickly with distance from roads compared to accumulation mode particle numbers.
2.1.2. Trends and Temporal Variability
Overall, PM 2.5 concentrations decreased from 1999 (the beginning of nationwide monitoring for PM 2.5 ) to 2007 in all ten EPA Regions, with the 3-yr avg of the 98th percentile of 24-h PM 2.5 concentrations dropping 10% over this time period. However from 2002-2007, concentrations of PM 2.5 were nearly constant with decreases observed in only some EPA Regions (Section 3.5.2.1). Concentrations of PM 2.5 components were only available for 2002-2007 using CSN data and showed little decline over this time period. This trend in PM 2.5 components is consistent with trends in PM 2.5 mass concentration observed after 2002 (shown in Figures 3-44 through 3-47). Concentrations of PM 10 also declined from 1988 to 2007 in all ten EPA Regions.
Using hourly PM observations in the 15 metropolitan areas, diel variation showed average hourly peaks that differ by size fraction and region (Section 3.5.2.3). For both PM 2.5 and PM 10 , a morning peak was typically observed starting at approximately 6:00 a.m., corresponding with the start of morning rush hour. There was also an evening concentration peak that was broader than the morning peak and extended into the overnight period, reflecting the concentration increase caused by the usual collapse of the mixing layer after sundown. The magnitude and duration of these peaks varied considerably by metropolitan area investigated.
UFPs were found to exhibit similar two-peaked diel patterns in Los Angeles and the San Joaquin Valley of CA and Rochester, NY as well as in Kawasaki City, Japan, and Copenhagen, Denmark. The morning peak in UFPs likely represents primary source emissions, such as rush-hour traffic, while the afternoon peak likely represents the combination of primary source emissions and nucleation of new particles.
2.1.3. Correlations between Copollutants
Correlations between PM and gaseous copollutants, including SO 2 , NO 2 , carbon monoxide (CO) and O 3 , varied both seasonally and spatially between and within metropolitan areas (Section 3.5.3). On average, PM 2.5 and PM 10 were correlated with each other better than with the gaseous copollutants. Although data are limited for PM 10-2.5 , the available data suggest a stronger correlation between PM 10 and PM 10-2.5 than between PM 2.5 and PM 10-2.5 on a national basis.There was relatively little seasonal variability in the mean correlation between PM in both size fractions and SO 2 and NO 2 . CO, however, showed higher correlations with PM 2.5 and PM 10 on average in the winter compared with the other seasons. This seasonality results in part because a larger fraction of PM is primary in origin during the winter. To the extent that this primary component of PM is associated with common combustion sources of NO 2 and CO, then higher correlations with these gaseous copollutants are to be expected. Increased atmospheric stability in colder months also results in higher correlations between primary pollutants (Section 3.5).
The correlation between daily maximum 8-h avg O 3 and 24-h avg PM 2.5 showed the highest degree of seasonal variability with positive correlations on average in summer (avg = 0.56) and negative correlations on average in the winter (avg = -0.30). During the transition seasons, spring and fall, correlations were mixed but on average were still positive. PM 2.5 is both primary and secondary in origin, whereas O 3 is only secondary. Photochemical production of O 3 and secondary PM in the planetary boundary layer (PBL) is much slower during the winter than during other seasons. Primary pollutant concentrations (e.g., primary PM 2.5 components, NO and NO 2 ) in many urban areas are elevated in winter as the result of heating emissions, cold starts and low mixing heights. O 3 in the PBL during winter is mainly associated with air subsiding from above the boundary layer following the passage of cold fronts, and this subsiding air has much lower PM concentrations than are present in the PBL. Therefore, a negative association between O 3 and PM 2.5 is frequently observed in the winter. During summer, both O 3 and secondary PM 2.5 are produced in the PBL and in the lower free troposphere at faster rates compared to winter, and so they tend to be positively correlated.
2.1.4. Measurement Techniques
The federal reference methods (FRMs) for PM 2.5 and PM 10 are based on criteria outlined in the Code of Federal Regulations. They are, however, subject to several limitations that should be kept in mind when using compliance monitoring data for health studies. For example, FRM techniques are subject to the loss of semi-volatile species such as organic compounds and ammonium nitrate (especially in the West). Since FRMs based on gravimetry use 24-h integrated filter samples to collect PM mass, no information is available for variations over shorter averaging times from these instruments. However, methods have been developed to measure real-time PM mass concentrations. Real-time (or continuous and semi-continuous) measurement techniques are also available for PM species, such as particle into liquid sampler (PILS) for multiple ions analysis and aerosol mass spectrometer (AMS) for multiple components analysis (Section 3.4.1). Advances have also been achieved in PM organic speciation. New 24-h FRMs and Federal Equivalent Methods (FEMs) based on gravimetry and continuous FEMs for PM 10-2.5 are available. FRMs for PM 10-2.5 rely on calculating the difference between co-located PM 10 and PM 2.5 measurements while a dichotomous sampler is designated as an FEM.
2.1.5. PM Formation in the Atmosphere and Removal
PM in the atmosphere contains both primary (i.e., emitted directly by sources) and secondary components, which can be anthropogenic or natural in origin. Secondary PM components can be produced by the oxidation of precursor gases such as SO 2 and NO X to acids followed by neutralization with ammonia (NH 3 ) and the partial oxidation of organic compounds. In addition to being emitted as primary particles, UFPs are produced by the nucleation of H 2 SO 4 vapor, H 2 O vapor, and perhaps NH 3 and certain organic compounds. Over most of the earth’s surface, nucleation is probably the major mechanism forming new UFPs. New UFP formation has been observed in environments ranging from relatively unpolluted marine and continental environments to polluted urban areas as an ongoing background process and during nucleation events. However, as noted above, a large percentage of UFPs come from combustion-related sources such as motor vehicles.
Developments in the chemistry of formation of secondary organic aerosol (SOA) indicate that oligomers are likely a major component of OC in aerosol samples. Recent observations also suggest that small but significant quantities of SOA are formed from the oxidation of isoprene in addition to the oxidation of terpenes and organic hydrocarbons with six or more carbon atoms. Gasoline engines have been found to emit a mix of nucleation-mode heavy and large polycyclic aromatic hydrocarbons on which unspent fuel and trace metals can condense, while diesel particles are composed of a soot nucleus on which sulfates and hydrocarbons can condense. To the extent that the primary component of organic aerosol is overestimated in emissions from combustion sources, the semi-volatile components are underestimated. This situation results from the lack of capture of evaporated semi-volatile components upon dilution in common emissions tests. As a result, near- traffic sources of precursors to SOA would be underestimated. The oxidation of these precursors results in more oxidized forms of SOA than previously considered, in both near source urban environments and further downwind. Primary organic aerosol can also be further oxidized to forms that have many characteristics in common with oxidized SOA formed from gaseous precursors. Organic peroxides constitute a significant fraction of SOA and represent an important class of reactive oxygen species (ROS) that have high oxidizing potential. More information on sources, emissions and deposition of PM are included in Section 3.3.
Wet and dry deposition are important processes for removing PM and other pollutants from the atmosphere on urban, regional, and global scales. Wet deposition includes incorporation of particles into cloud droplets that fall as rain (rainout) and collisions with falling rain (washout). Other hydrometeors (snow, ice) can also serve the same purpose. Dry deposition involves transfer of particles through gravitational settling and/or by impaction on surfaces by turbulent motions. The effects of deposition of PM on ecosystems and materials are discussed in Section 2.5 and in Chapter 9.
2.1.6. Source Contributions to PM
Results of receptor modeling calculations indicate that PM 2.5 is produced mainly by combustion of fossil fuel, either by stationary sources or by transportation. A relatively small number of broadly defined source categories, compared to the total number of chemical species that typically are measured in ambient monitoring source receptor studies, account for the majority of the observed PM mass. Some ambiguity is inherent in identifying source categories. For example, quite different mobile sources such as trucks, farm equipment, and locomotives rely on diesel engines and ancillary data is often required to resolve these sources. A compilation of study results shows that secondary SO 4 2– (derived mainly from SO 2 emitted by Electricity Generating Units [EGUs]), NO 3 – (from the oxidation of NO x emitted mainly from transportation sources and EGUs), and primary mobile source categories, constitute most of PM 2.5 (and PM 10 ) in the East. PM 10-2.5 is mainly primary in origin, having been emitted as fully formed particles derived from abrasion and crushing processes, soil disturbances, plant and insect fragments, pollens and other microorganisms, desiccation of marine aerosol emitted from bursting bubbles, and hygroscopic fine PM expanding with humidity to coarse mode. Gases such as HNO 3 can also condense directly onto preexisting coarse particles. Suspended primary coarse PM can contain Fe, Si, Al, and base cations from soil, plant and insect fragments, pollen, fungal spores, bacteria, and viruses, as well as fly ash, brake lining particles, debris, and automobile tire fragments. Quoted uncertainties in the source apportionment of constituents in ambient aerosol samples typically range from 10 to 50%. An intercomparison of source apportionment techniques indicated that the same major source categories of PM 2.5 were consistently identified by several independent groups working with the same data sets. Soil-, sulfate-, residual oil-, and salt-associated mass were most clearly identified by the groups. Other sources with more ambiguous signatures, such as vegetative burning and traffic-related emissions were less consistently identified.
Spatial variability in source contributions across urban areas is an important consideration in assessing the likelihood of exposure error in epidemiologic studies relating health outcomes to sources. Concepts similar to those for using ambient concentrations as surrogates for personal exposures apply here. Some source attribution studies for PM 2.5 indicate that intra-urban variability increases in the following order: regional sources (e.g., secondary SO 4 2– originating from EGUs) < area sources (e.g., on-road mobile sources) < point sources (e.g., metals from stacks of smelters).
Although limited information was available for PM 10-2.5 , it does indicate a similar ordering, but without a regional component (resulting from the short lifetime of PM 10-2.5 compared to transport times on the regional scale). More discussion on source contributions to PM is available in Section 3.6.
2.1.7. Policy-Relevant Background
The background concentrations of PM that are useful for risk and policy assessments, which inform decisions about the NAAQS are referred to as policy-relevant background (PRB) concentrations. PRB concentrations have historically been defined by EPA as those concentrations that would occur in the U.S. in the absence of anthropogenic emissions in continental North America defined here as the U.S., Canada, and Mexico. For this document, PRB concentrations include contributions from natural sources everywhere in the world and from anthropogenic sources outside continental North America. Background concentrations so defined facilitated separation of pollution that can be controlled by U.S. regulations or through international agreements with neighboring countries from those that were judged to be generally uncontrollable by the U.S. Over time, consideration of potential broader ranging international agreements may lead to alternative determinations of which PM source contributions should be considered by EPA as part of PRB.
Contributions to PRB concentrations of PM include both primary and secondary natural and anthropogenic components. For this document, PRB concentrations of PM 2.5 for the continental U.S. were estimated using EPA’s Community Multi-scale Air Quality (CMAQ) modeling system, a deterministic, chemical-transport model (CTM), using output from GEOS-Chem a global-scale model for CMAQ boundary conditions. PRB concentrations of PM 2.5 were estimated to be less than 1 μg/m3 on an annual basis, with maximum daily average values in a range from 3.1 to 20 μg/m3 and having a peak of 63 μg/m3 at the nine national park sites across the U.S. used to evaluate model performance for this analysis. A description of the models and evaluation of their performance is given in Section 3.6 and further details about the calculations of PRB concentrations are given in Section 3.7.
2.2. Human Exposure
This section summarizes the findings from the recent exposure assessment literature. This summary is intended to support the interpretation of the findings from epidemiologic studies and reflects the material presented in Section 3.8. Attention is given to how concentration metrics can be used in exposure assessment and what errors and uncertainties are incurred for different approaches. Understanding of exposure errors is important because exposure error can potentially bias an estimate of a health effect or increase the size of confidence intervals around a health effect estimate. 2.2.1. Spatial Scales of PM Exposure Assessment
Assessing population-level exposure at the urban scale is particularly relevant for time-series epidemiologic studies, which provide information on the relationship between health effects and community-average exposure, rather than an individual’s exposure. PM concentrations measured at a central-site ambient monitor are used as surrogates for personal PM exposure. However, the correlation between the PM concentration measured at central-site ambient monitor(s) and the unknown true community average concentration depends on the spatial distribution of PM, the location of the monitoring site(s) chosen to represent the community average, and division of the community by terrain features or local sources into several sub-communities that differ in the temporal pattern of pollution. Concentrations of SO 4 2– and some components of SOA measured at central-site monitors are expected to be uniform in urban areas because of the regional nature of their sources. However, this is not true for primary components like EC whose sources are strongly spatially variable in urban areas.
At micro-to-neighborhood scales, heterogeneity of sources and topography contribute to variability in exposure. This is particularly true for PM 10-2.5 and for UFPs, which have spatially variable urban sources and loss processes (mainly gravitational settling for PM 10-2.5 and coagulation for UFPs) that also limit their transport from sources more readily than for PM 2.5 . Personal activity patterns also vary across urban areas and across regions. Some studies, conducted mainly in Europe, have found personal PM 2.5 and PM 10 exposures for pedestrians in street canyons to be higher than ambient concentrations measured by urban central site ambient monitors. Likewise, microenvironmental UFP concentrations were observed to be substantially higher in near-road environments, street canyons, and tunnels when compared with urban background concentrations. In-vehicle UFP and PM 2.5 exposures can also be important. As a result, concentrations measured by ambient monitors likely do not reflect the contributions of UFP or PM 2.5 exposures to individuals while commuting.
There is significant variability within and across regions of the country with respect to indoor exposures to ambient PM. Infiltrated ambient PM concentrations depend in part on the ventilation properties of the building or vehicle in which the person is exposed. PM infiltration factors depend on particle size, chemical composition, season, and region of the country. Infiltration can best be modeled dynamically rather than being represented by a single value. Season is important to PM infiltration because it affects the ventilation practices (e.g., open windows) used. In addition, ambient temperature and humidity conditions affect the transport, dispersion, and size distribution of PM. Residential air exchange rates have been observed to be higher in the summer for regions with low air conditioning usage. Regional differences in air exchange rates (Southwest < Southeast < Northeast < Northwest) also reflect ventilation practices. Differential infiltration occurs as a function of PM size and composition (the latter of which is described below). PM infiltration is larger for accumulation mode particles than for UFPs and PM 10-2.5 . Differential infiltration by size fraction can affect exposure estimates if not accurately characterized.
2.2.2. Exposure to PM Components and Copollutants
Emission inventories and source apportionment studies suggest that sources of PM exposure vary by region. Comparison of studies performed in the eastern U.S. with studies performed in the western U.S. suggest that the contribution of SO 4 2– to exposure is higher for the East (16-46%) compared with the West (~4%) and that motor vehicle emissions and secondary NO 3 – are larger sources of exposure for the West (~9%) as compared with the East (~4%). Results of source apportionment studies of exposure to SO 4 2– indicate that SO 4 2– exposures are mainly attributable to ambient sources. Source apportionment for OC and EC is difficult because they originate from both indoor and outdoor sources. Exposure to OC of indoor and outdoor origin can be distinguished by the presence of aliphatic C-H groups generated indoors, since outdoor concentrations of aliphatic C-H are low. Studies of personal exposure to ambient trace metal have shown significant variation among cities and over seasons. This is in response to geographic and seasonal variability in sources including incinerator operation, fossil fuel combustion, biomass combustion (wildfires), and the resuspension of crustal materials in the built environment. Differential infiltration is also affected by variations in particle composition and volatility. For example, EC infiltrates more readily than OC. This can lead to outdoor-indoor differentials in PM composition.
Some studies have explored the relationship between PM and copollutant gases and suggested that certain gases can serve as surrogates for describing exposure to other air pollutants. The findings indicate that ambient concentrations of gaseous copollutants can act as surrogates for personal exposure to ambient PM. Several studies have concluded that ambient concentrations of O 3 , NO 2 , and SO 2 are associated with the ambient component of personal exposure to total PM 2.5 . If associations between ambient gases and personal exposure to PM 2.5 of ambient origin exist, such associations are complex and vary by season and location. 2.2.3. Implications for Epidemiologic Studies
In epidemiologic studies, exposure may be estimated using various approaches, most of which rely on measurements obtained using central site monitors. The magnitude and direction of the biases introduced through error in exposure measurement depend on the extent to which the error is associated with the measured PM concentration. In general, when exposure error is not strongly correlated with the measured PM concentration, bias is toward the null and effect estimates are underestimated. Moreover, lack of information regarding exposure measurement error can also add uncertainty to the health effects estimate.
One important factor to be considered is the spatial variation in PM concentrations. The degree of urban-scale spatial variability in PM concentrations varies across the country and by size fraction. PM 2.5 concentrations are relatively well-correlated across monitors in the urban areas examined for this assessment. The limited available evidence indicates that there is greater spatial variability in PM 10-2.5 concentrations than PM 2.5 concentrations, resulting in increased exposure error for the larger size fraction. Likewise, studies have shown UFPs to be more spatially variable across urban areas compared to PM 2.5 . Even if PM 2.5 , PM 10-2.5 , or UFP concentrations measured at sites within an urban area are generally highly correlated, significant spatial variation in their concentrations can occur on any given day. In addition, there can be differential exposure errors for PM components (e.g., SO 4 2–, OC, EC). Current information suggests that UFPs, PM 10-2.5, and some PM components are more spatially variable than PM 2.5 . Spatial variability of these PM indicators adds uncertainty to exposure estimates.
Overall, recent studies generally confirm and build upon the key conclusions of the 2004 PM AQCD: separation of total PM exposures into ambient and nonambient components reduces potential uncertainties in the analysis and interpretation of PM health effects data; and ambient PM concentration can be used as a surrogate for ambient PM exposure in community time-series epidemiologic studies because the change in ambient PM concentration should be reflected in the change in the health risk coefficient. The use of the community average ambient PM 2.5 concentration as a surrogate for the community average personal exposure to ambient PM 2.5 is not expected to change the principal conclusions from time-series and most panel epidemiologic studies that use community average health and pollution data. Several recent studies support this by showing how the ambient component of personal exposure to PM 2.5 could be estimated using various tracer and source apportionment techniques and by showing that the ambient component is highly correlated with ambient concentrations of PM 2.5 . These studies show that the non-ambient component of personal exposure to PM 2.5 is largely uncorrelated with ambient PM 2.5 concentrations. A few panel epidemiologic studies have included personal as well as ambient monitoring data, and generally reported associations with all types of PM measurements. Epidemiologic studies of long-term exposure typically exploit the differences in PM concentration across space, as well as time, to estimate the effect of PM on the health outcome of interest. Long-term exposure estimates are most accurate for pollutants that do not vary substantially within the geographic area studied.
2.3. Health Effects
This section evaluates the evidence from toxicological, controlled human exposure, and epidemiologic studies that examined the health effects associated with short- and long-term exposure to PM (i.e., PM 2.5 , PM 10-2.5 and UFPs). The results from the health studies evaluated in combination with the evidence from atmospheric chemistry and exposure assessment studies contribute to the causal determinations made for the health outcomes discussed in this assessment (a description of the causal framework can be found in Section 1.5.4). In the following sections a discussion of the causal determinations will be presented by PM size fraction and exposure duration (i.e., short- or long-term exposure) for the health effects for which sufficient evidence was available to conclude a causal, likely to be causal or suggestive relationship. Although not presented in depth in this chapter, a detailed discussion of the underlying evidence used to formulate each causal determination can be found in Chapters 6 and 7.
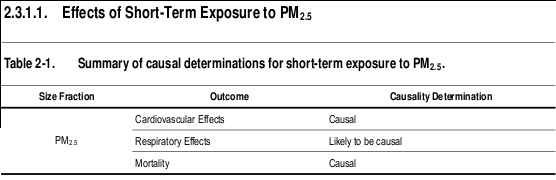
Cardiovascular Effects
Epidemiologic studies that examined the effect of PM 2.5 on cardiovascular emergency department (ED) visits and hospital admissions reported consistent positive associations (predominantly for ischemic heart disease [IHD] and congestive heart failure [CHF]), with the majority of studies reporting increases ranging from 0.5 to 3.4% per 10 μg/m3 increase in PM 2.5 . These effects were observed in study locations with mean 1 24-h avg PM 2.5 concentrations ranging from 7-18 μg/m3 (Section 6.2.10). The largest U.S.-based multicity study evaluated, Medicare Air Pollution Study (MCAPS), provided evidence of regional heterogeneity (e.g., the largest excess risks occurred in the Northeast [1.08%]) and seasonal variation (e.g., the largest excess risks occurred during the winter season [1.49%]) in PM 2.5 cardiovascular disease (CVD) risk estimates, which is consistent with the null findings of several single-city studies conducted in the western U.S. These associations are supported by multicity epidemiologic studies that observed consistent positive associations between short-term exposure to PM 2.5 and cardiovascular mortality and also reported regional and seasonal variability in risk estimates. The multicity studies evaluated reported consistent increases in cardiovascular mortality ranging from 0.47 to 0.85% in study locations with mean 24-h avg PM 2.5 concentrations above 12.8 μg/m3 (Table 6-15).
Controlled human exposure studies have demonstrated PM 2.5 -induced changes in various measures of cardiovascular function among healthy and health-compromised adults. The most consistent evidence is for altered vasomotor function following exposure to diesel exhaust (DE) or CAPs with O 3 (Section 6.2.4.2). Although these findings provide biological plausibility for the observations from epidemiologic studies, the fresh DE used in the controlled human exposure studies evaluated contains gaseous components (e.g., CO, NO x ), and therefore, the possibility that some of the changes in vasomotor function might be due to gaseous components cannot be ruled out. Furthermore, the prevalence of UFPs in fresh DE limits the ability to conclusively attribute the observed effects to either the UF fraction or PM 2.5 as a whole. An evaluation of toxicological studies found evidence for altered vessel tone and microvascular reactivity, which provide coherence and biological plausibility for the vasomotor effects that have been observed in both the controlled human exposure and epidemiologic studies (Section 6.2.4.3). However, most of these toxicological studies exposed animals via intratracheal (IT) instillation or using relatively high inhalation concentrations.
In addition to the effects observed on vasomotor function, myocardial ischemia has been observed across disciplines through PM 2.5 effects on ST-segment depression, with toxicological studies providing biological plausibility by demonstrating reduced blood flow during ischemia (Section 6.2.3). There is also a growing body of evidence from controlled human exposure and toxicological studies demonstrating PM 2.5 -induced changes on heart rate variability (HRV) and markers of systemic oxidative stress (Sections 6.2.1 and 6.2.9, respectively). Additional but inconsistent effects of PM 2.5 on blood pressure (BP), blood coagulation markers, and markers of systemic inflammation have also been reported across disciplines. Toxicological studies have provided biologically plausible mechanisms (e.g., increased right ventricular pressure and diminished cardiac contractility) for the associations observed between PM 2.5 and CHF in epidemiologic studies.
Together, the collective evidence from epidemiologic, controlled human exposure, and toxicological studies is sufficient to conclude that a causal relationship exists between short- term exposures to PM 2.5 and cardiovascular effects.
Respiratory Effects
The recent epidemiologic studies evaluated report consistent positive associations between short-term exposure to PM 2.5 and respiratory ED visits and hospital admissions for chronic obstructive pulmonary disease (COPD) and respiratory infections (Section 6.3). Positive associations were also observed for asthma ED visits and hospital admissions for adults and children combined, but effect estimates are imprecise and not consistently positive for children alone. Most studies reported effects in the range of ~1% to 4% increase in respiratory hospital admissions and ED visits and were observed in study locations with mean 24-h avg PM 2.5 concentrations ranging from 6.1-22 μg/m3. Additionally, multicity epidemiologic studies reported consistent positive associations between short-term exposure to PM 2.5 and respiratory mortality as well as regional and seasonal variability in risk estimates. The multicity studies evaluated reported consistent, precise increases in respiratory mortality ranging from 1.67 to 2.20% in study locations with mean 24-h avg PM 2.5 concentrations above 12.8 μg/m3 (Table 6-15). Evidence for PM 2.5 -related respiratory effects was also observed in panel studies, which indicate associations with respiratory symptoms, pulmonary function, and pulmonary inflammation among asthmatic children. Although not consistently observed, some controlled human exposure studies have reported small decrements in various measures of pulmonary function following controlled exposures to PM 2.5 (Section 6.3.2.2).
Controlled human exposure studies using adult volunteers have demonstrated increased markers of pulmonary inflammation following exposure to a variety of different particle types; oxidative responses to DE and wood smoke; and exacerbations of allergic responses and allergic sensitization following exposure to DE particles (Section 6.3). Toxicological studies have provided additional support for PM 2.5 -related respiratory effects through inhalation exposures of animals to CAPs, DE, other traffic-related PM and wood smoke. These studies reported an array of respiratory effects including altered pulmonary function, mild pulmonary inflammation and injury, oxidative responses, airway hyperresponsiveness (AHR) in allergic and non-allergic animals, exacerbations of allergic responses, and increased susceptibility to infections (Section 6.3).
Overall, the evidence for an effect of PM 2.5 on respiratory outcomes is somewhat restricted by limited coherence between some of the findings from epidemiologic and controlled human exposure studies for the specific health outcomes reported and the sub-populations in which those health outcomes occur. Epidemiologic studies have reported variable results among specific respiratory outcomes, specifically in asthmatics (e.g., increased respiratory symptoms in asthmatic children, but not increased asthma hospital admissions and ED visits) (Section 6.3.8). Additionally, respiratory effects have not been consistently demonstrated following controlled exposures to PM 2.5 among asthmatics or individuals with COPD. Collectively, the epidemiologic, controlled human exposure, and toxicological studies evaluated demonstrate a wide range of respiratory responses, and although results are not fully consistent and coherent across studies the evidence is sufficient to conclude that a causal relationship is likely to exist between short-term exposures to PM 2.5 and respiratory effects.
Mortality
An evaluation of the epidemiologic literature indicates consistent positive associations between short-term exposure to PM 2.5 and all-cause, cardiovascular-, and respiratory-related mortality (Section 6.5.2.2.). The evaluation of multicity studies found that consistent and precise risk estimates for all-cause (nonaccidental) mortality that ranged from 0.29 to 1.21% per 10 μg/m3 increase in PM 2.5 at lags of 1 and 0-1 days. In these study locations, mean 24-h avg PM 2.5 concentrations were 12.8 μg/m3 and above (Table 6-15). Cardiovascular-related mortality risk estimates were found to be similar to those for all-cause mortality; whereas, the risk estimates for respiratory-related mortality were consistently larger (i.e., 1.01-2.2%) using the same lag periods and averaging indices. The studies evaluated that examined the relationship between short-term exposure to PM 2.5 and cardiovascular effects (Section 6.2) provide coherence and biological plausibility for PM 2.5 -induced cardiovascular mortality, which represents the largest component of total (nonaccidental) mortality (~ 35%) (American Heart Association, 2009, 198920). However, as noted in Section 6.3, there is limited coherence between some of the respiratory morbidity findings from epidemiologic and controlled human exposure studies for the specific health outcomes reported and the subpopultions in which those health outcomes occur, complicating the interpretation of the PM 2.5 respiratory mortality effects observed. Regional and seasonal patterns in PM 2.5 risk estimates were observed with the greatest effect estimates occurring in the eastern U.S. and during the spring. Of the studies evaluated only Burnett et al. (2004, 086247), a Canadian multicity study, analyzed gaseous pollutants and found mixed results, with possible confounding of PM 2.5 risk estimates by NO 2 . Although the recently evaluated U.S.-based multicity studies did not analyze potential confounding of PM 2.5 risk estimates by gaseous pollutants, evidence from the limited number of single-city studies evaluated in the 2004 PM AQCD (U.S. EPA, 2004, 056905) suggest that gaseous copollutants do not confound the PM 2.5 -mortality association. This is further supported by studies that examined the PM 10 -mortality relationship. An examination of effect modifiers (e.g., demographic and socioeconomic factors), specifically air conditioning use as an indicator for decreased pollutant penetration indoors, has suggested that PM 2.5 risk estimates increase as the percent of the population with access to air conditioning decreases. Collectively, the epidemiologic literature provides evidence that a causal relationship exists between short-term exposures to PM 2.5 and mortality.
2.3.1.2. Effects of Long-Term Exposure to PM 2.5
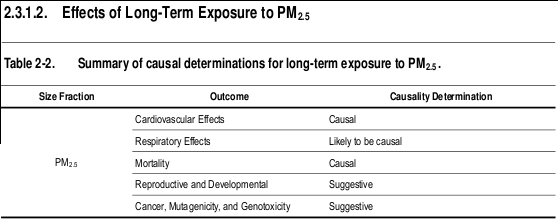
Cardiovascular Effects
The strongest evidence for cardiovascular health effects related to long-term exposure to PM 2.5 comes from large, multicity U.S.-based studies, which provide consistent evidence of an association between long-term exposure to PM 2.5 and cardiovascular mortality (Section 7.2.10). These associations are supported by a large U.S.-based epidemiologic study (i.e., Women’s Health Initiative [WHI] study) that reports associations between PM 2.5 and CVDs among post-menopausal women using a 1-yr avg PM 2.5 concentration (mean = 13.5 μg/m3) (Section 7.2). However, epidemiologic studies that examined subclinical markers of CVD report inconsistent findings. Epidemiologic studies have also provided some evidence for potential modification of the PM 2.5 -CVD association when examining individual-level data, specifically smoking status and the use of anti- hyperlipidemics. Although epidemiologic studies have not consistently detected effects on markers of atherosclerosis due to long-term exposure to PM 2.5 , toxicological studies have provided strong evidence for accelerated development of atherosclerosis in ApoE-/- mice exposed to CAPs and have shown effects on coagulation, experimentally-induced hypertension, and vascular reactivity (Section 7.2.1.2). Evidence from toxicological studies provides biological plausibility and coherence with studies of short-term exposure and cardiovascular morbidity and mortality, as well as with studies that examined long-term exposure to PM 2.5 and cardiovascular mortality. Taken together, the evidence from epidemiologic and toxicological studies is sufficient to conclude that a causal relationship exists between long-term exposures to PM 2.5 and cardiovascular effects.
Respiratory Effects
Recent epidemiologic studies conducted in the U.S. and abroad provide evidence of associations between long-term exposure to PM 2.5 and decrements in lung function growth, increased respiratory symptoms, and asthma development in study locations with mean PM 2.5 concentrations ranging from 13.8 to 30 μg/m3 during the study periods (Section 7.3.1.1 and Section 7.3.2.1). These results are supported by studies that observed associations between long-term exposure to PM 10 and an increase in respiratory symptoms and reductions in lung function growth in areas where PM 10 is dominated by PM 2.5 . However, the evidence to support an association with long-term exposure to PM 2.5 and respiratory mortality is limited (Figure 7-7). Subchronic and chronic toxicological studies of CAPs, DE, roadway air and woodsmoke provide coherence and biological plausibility for the effects observed in the epidemiologic studies. These toxicological studies have presented some evidence for altered pulmonary function, mild inflammation, oxidative responses, immune suppression, and histopathological changes including mucus cell hyperplasia (Section 7.3). Exacerbated allergic responses have been demonstrated in animals exposed to DE and wood smoke. In addition, pre- and postnatal exposure to ambient levels of urban particles was found to affect lung development in an animal model. This finding is important because impaired lung development is one mechanism by which PM exposure may decrease lung function growth in children. Collectively, the evidence from epidemiologic and toxicological studies is sufficient to conclude that a causal relationship is likely to exist between long-term exposures to PM 2.5 and respiratory effects.
Mortality
The recent epidemiologic literature reports associations between long-term PM 2.5 exposure and increased risk of mortality. Mean PM 2.5 concentrations ranged from 13.2 to 29 μg/m3 during the study period in these areas (Section 7.6). When evaluating cause-specific mortality, the strongest evidence can be found when examining associations between PM 2.5 and cardiovascular mortality, and positive associations were also reported between PM 2.5 and lung cancer mortality (Figure 7-7). The cardiovascular mortality association has been confirmed further by the extended Harvard Six Cities and American Cancer Society studies, which both report strong associations between long- term exposure to PM 2.5 and cardiopulmonary and IHD mortality (Figure 7-7). Additional new evidence from a study that used the WHI cohort found a particularly strong association between long-term exposure to PM 2.5 and CVD mortality in post-menopausal women. Fewer studies have evaluated the respiratory component of cardiopulmonary mortality, and, as a result, the evidence to support an association with long-term exposure to PM 2.5 and respiratory mortality is limited (Figure 7-7). The evidence for cardiovascular and respiratory morbidity due to short- and long-term exposure to PM 2.5 provides biological plausibility for cardiovascular- and respiratory-related mortality. Collectively, the evidence is sufficient to conclude that a causal relationship exists between long-term exposures to PM 2.5 and mortality.
Reproductive and Developmental Effects
Evidence is accumulating for PM 2.5 effects on low birth weight and infant mortality, especially due to respiratory causes during the post-neonatal period. The mean PM 2.5 concentrations during the study periods ranged from 5.3-27.4 μg/m3 (Section 7.4), with effects becoming more precise and consistently positive in locations with mean PM 2.5 concentrations of 15 μg/m3 and above (Section 7.4). Exposure to PM 2.5 was usually associated with greater reductions in birth weight than exposure to PM 10 . The evidence from a few U.S. studies that investigated PM 10 effects on fetal growth, which reported similar decrements in birth weight, provide consistency for the PM 2.5 associations observed and strengthen the interpretation that particle exposure may be causally related to reductions in birth weight. The epidemiologic literature does not consistently report associations between long-term exposure to PM and preterm birth, growth restriction, birth defects or decreased sperm quality. Toxicological evidence supports an association between PM 2.5 and PM 10 exposure and adverse reproductive and developmental outcomes, but provide little mechanistic information or biological plausibility for an association between long-term PM exposure and adverse birth outcomes (e.g., low birth weight or infant mortality). New evidence from animal toxicological studies on heritable mutations is of great interest, and warrants further investigation. Overall, the epidemiologic and toxicological evidence is suggestive of a causal relationship between long- term exposures to PM 2.5 and reproductive and developmental outcomes.
Cancer, Mutagenicity, and Genotoxicity
Multiple epidemiologic studies have shown a consistent positive association between PM 2.5 and lung cancer mortality, but studies have generally not reported associations between PM 2.5 and lung cancer incidence (Section 7.5). Animal toxicological studies have examined the potential relationship between PM and cancer, but have not focused on specific size fractions of PM. Instead they have examined ambient PM, wood smoke, and DEP. A number of studies indicate that ambient urban PM, emissions from wood/biomass burning, emissions from coal combustion, and gasoline and DE are mutagenic, and that PAHs are genotoxic. These findings are consistent with earlier studies that concluded that ambient PM and PM from specific combustion sources are mutagenic and genotoxic and provide biological plausibility for the results observed in the epidemiologic studies. A limited number of epidemiologic and toxicological studies examined epigenetic effects, and demonstrate that PM induces some changes in methylation. However, it has yet to be determined how these alterations in the genome could influence the initiation and promotion of cancer. Additionally, inflammation and immune suppression induced by exposure to PM may confer susceptibility to cancer. Collectively, the evidence from epidemiologic studies, primarily those of lung cancer mortality, along with the toxicological studies that show some evidence of the mutagenic and genotoxic effects of PM is suggestive of a causal relationship between long-term exposures to PM 2.5 and cancer.
2.3.2. Integration of PM 2.5 Health Effects
In epidemiologic studies, short-term exposure to PM 2.5 is associated with a broad range of respiratory and cardiovascular effects, as well as mortality. For cardiovascular effects and mortality, the evidence supports the existence of a causal relationship with short-term PM 2.5 exposure; while the evidence indicates that a causal relationship is likely to exist between short-term PM 2.5 exposure and respiratory effects. The effect estimates from recent and older U.S. and Canadian-based epidemiologic studies that examined the relationship between short-term exposure to PM 2.5 and health outcomes with mean 24-h avg PM 2.5 concentrations <17 μg/m3 are shown in Figure 2-1. A number of different health effects are included in Figure 2-1 to provide an integration of the range of effects by mean concentration, with a focus on cardiovascular and respiratory effects and all-cause (nonaccidental) mortality (i.e., health effects categories with at least a suggestive causal determination). A pattern of consistent positive associations with mortality and morbidity effects can be seen in this figure. Mean PM 2.5 concentrations ranged from 6.1 to 16.8 μg/m3.in these study locations.
Figure 2-1: Summary of effect estimates (per 10 μg/m3) by increasing concentration from U.S. studies examining the association between short-term exposure to PM 2.5 and cardiovascular and respiratory effects, and mortality, conducted in locations where the reported mean 24-h avg PM 2.5 concentrations were <17 μg/m3.
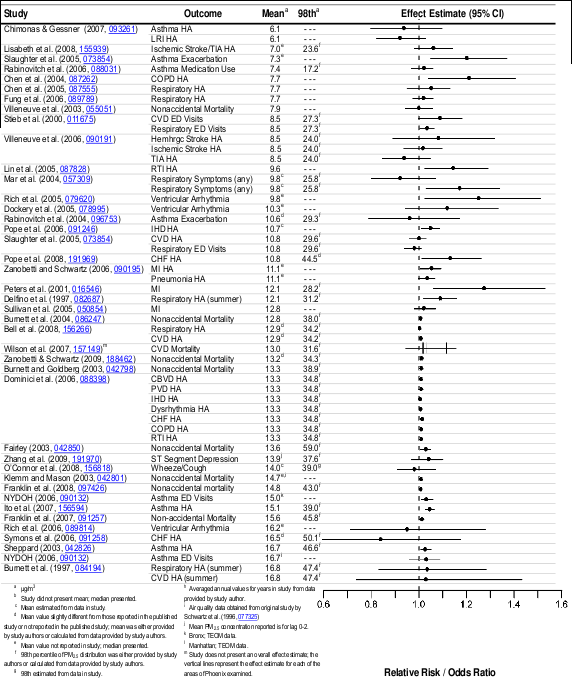
Long-term exposure to PM 2.5 has been associated with health outcomes similar to those found in the short-term exposure studies, specifically for respiratory and cardiovascular effects and mortality. As found for short-term PM 2.5 exposure, the evidence indicates that a causal relationship exists between long-term PM 2.5 exposure and cardiovascular effects and mortality, and that a causal relationship is likely to exist between long-term PM 2.5 exposure and effects on the respiratory system.
Figure 2-2 highlights the findings of epidemiologic studies where the long-term mean PM 2.5 concentrations were ≤ 29 μg/m3. A range of health outcomes are displayed (including cardiovascular mortality, all-cause mortality, infant mortaltiy, and bronchitis) ordered by mean concentration. The range of mean PM 2.5 concentrations in these studies was 10.7-29 μg/m3 during the study periods. Additional studies not included in this figure that focus on subclinical outcomes, such as changes in lung function or atherosclerotic markers also report effects in areas with similar concentrations (Sections 7.2 and 7.3). Although not highlighted in the summary figure, long-term PM 2.5 exposure studies also provide evidence for reproductive and developmental effects (i.e., low birth weight) and cancer (i.e., lung cancer mortality) in response to to exposure to PM 2.5.
Figure 2-2: Summary of effect estimates (per 10 μg/m3) by increasing concentration from U.S. studies examining the association between long-term exposure to PM 2.5 and cardiovascular and respiratory effects, and mortality.

The observations from both the short- and long-term exposure studies are supported by experimental findings of PM 2.5 -induced subclinical and clinical cardiovascular effects. Epidemiologic studies have shown an increase in ED visits and hospital admissions for IHD upon exposure to PM 2.5 . These effects are coherent with the changes in vasomotor function and ST- segment depression observed in both toxicological and controlled human exposure studies. It has been postulated that exposure to PM 2.5 can lead to myocardial ischemia through an effect on the autonomic nervous system or by altering vasomotor function. PM-induced systemic inflammation, oxidative stress and/or endothelial dysfunction may contribute to altered vasomotor function. These effects have been demonstrated in recent animal toxicological studies, along with altered microvascular reactivity, altered vessel tone, and reduced blood flow during ischemia. Toxicological studies demonstrating increased right ventricular pressure and diminished cardiac contractility also provide biological plausibility for the associations observed between PM 2.5 and CHF in epidemiologic studies.
Thus, the overall evidence from the short-term epidemiologic, controlled human exposure, and toxicological studies evaluated provide coherence and biological plausibility for cardiovascular effects related to myocardial ischemia and CHF. Coherence in the cardiovascular effects observed can be found in long-term exposure studies, especially for CVDs among post-menopausal women. Additional studies provide limited evidence for subclinical measures of atherosclerosis in epidemiologic studies with stronger evidence from toxicological studies that have demonstrated accelerated development of atherosclerosis in ApoE-/- mice exposed to PM 2.5 CAPs along with effects on coagulation, experimentally-induced hypertension, and vascular reactivity. Repeated acute responses to PM may lead to cumulative effects that manifest as chronic disease, such as atherosclerosis. Contributing factors to atherosclerosis development include systemic inflammation, endothelial dysfunction, and oxidative stress all of which are associated with PM 2.5 exposure. However, it has not yet been determined whether PM initiates or promotes atherosclerosis. The evidence from both short- and long-term exposure studies on cardiovascular morbidity provide coherence and biological plausibility for the cardiovascular mortality effects observed when examining both exposure durations. In addition, cardiovascular hospital admission and mortality studies that examined the PM 10 concentration-response relationship found evidence of a log-linear no-threshold relationship between PM exposure and cardiovascular-related morbidity (Section 6.2) and mortality (Section 6.5).
Epidemiologic studies have also reported respiratory effects related to short-term exposure to PM 2.5 , which include increased ED visits and hospital admissions, as well as alterations in lung function and respiratory symptoms in asthmatic children. These respiratory effects were found to be generally robust to the inclusion of gaseous pollutants in copollutant models with the strongest evidence from the higher powered studies (Figure 6-9 and Figure 6-15). Consistent positive associations were also reported between short-term exposure to PM 2.5 and respiratory mortality in epidemiologic studies. However, uncertainties exist in the PM 2.5 -respiratory mortality associations reported due to the limited number of studies that examined potential confounders of the PM 2.5 - respiratory mortality relationship, and the limited information regarding the biological plausibility of the clinical and subclinical respiratory outcomes observed in the epidemiologic and controlled human exposure studies (Section 6.3) resulting in the progression to PM 2.5 -induced respiratory mortality. Important new findings, which support the PM 2.5 -induced respiratory effects mentioned above, include associations with post-neonatal (between 1 mo and 1 yr of age) respiratory mortality. Controlled human exposure studies provide some support for the respiratory findings from epidemiologic studies, with demonstrated increases in pulmonary inflammation following short-term exposure. However, there is limited and inconsistent evidence of effects in response to controlled exposures to PM 2.5 on respiratory symptoms or pulmonary function among healthy adults or adults with respiratory disease. Long-term exposure epidemiologic studies provide additional evidence for PM 2.5 -induced respiratory morbidity, but little evidence for an association with respiratory mortality. These epidemiologic morbidity studies have found decrements in lung function growth, as well as increased respiratory symptoms, and asthma. Toxicological studies provide coherence and biological plausibility for the respiratory effects observed in response to short and long-term exposures to PM by demonstrating a wide array of biological responses including: altered pulmonary function, mild pulmonary inflammation and injury, oxidative responses, and histopathological changes in animals exposed by inhalation to PM 2.5 derived from a wide variety of sources. In some cases, prolonged exposures led to adaptive responses. Important evidence was also found in an animal model for altered lung development following pre- and post-natal exposure to urban air, which may provide a mechanism to explain the reduction in lung function growth observed in children in response to long-term exposure to PM.
Additional respiratory-related effects have been tied to allergic responses. Epidemiologic studies have provided evidence for increased hospital admissions for allergic symptoms (e.g., allergic rhinitis) in response to short- and long-term exposure to PM 2.5 . Panel studies also positively associate long-term exposure to PM 2.5 and PM 10 with indicators of allergic sensitization. Controlled human exposure and toxicological studies provide coherence for the exacerbation of allergic symptoms, by showing that PM 2.5 can promote allergic responses and intensify existing allergies. Allergic responses require repeated exposures to antigen over time and co-exposure to an adjuvant (possibly DE particles or UF CAPs) can enhance this response. Allergic sensitization often underlies allergic asthma, characterized by inflammation and AHR. In this way, repeated or chronic exposures involving multifactorial responses (immune system activation, oxidative stress, inflammation) can lead to irreversible outcomes. Epidemiologic studies have also reported evidence for increased hospital admissions for respiratory infections in response to both short- and long-term exposures to PM 2.5 . Toxicological studies suggest that PM impairs innate immunity, which is the first line of defense against infection, providing coherence for the respiratory infection effects observed in epidemiologic studies.
The difference in effects observed across studies and between cities may be attributed, at least in part, to the differences in PM composition across the U.S. Differences in PM toxicity may result from regionally varying PM composition and size distribution, which in turn reflects differences in sources and PM volatility. A person’s exposure to ambient PM will also vary due to regional differences in personal activity patterns, microenvironmental characteristics and the spatial variability of PM concentrations in urban areas. Regional differences in PM 2.5 composition are outlined briefly in Section 2.1 above and in more detail in Section 3.5. An examination of data from the CSN indicates that East-West gradients exist for a number of PM components. Specifically, SO 4 2- concentrations are higher in the East, OC constitutes a larger fraction of PM in the West, and NO 3 - concentrations are highest in the valleys of central California and during the winter in the Midwest. However, the available evidence and the limited amount of city-specific speciated PM 2.5 data does not allow conclusions to be drawn that specifically differentiate effects of PM in different locations.
It remains a challenge to determine relationships between specific constituents, combinations of constituents, or sources of PM 2.5 and the various health effects observed. Source apportionment studies of PM 2.5 have attempted to decipher some of these relationships and in the process have identified associations between multiple sources and various respiratory and cardiovascular health effects, as well as mortality. Although different source apportionment methods have been used across these studies, the methods used have been evaluated and found generally to identify the same sources and associations between sources and health effects (Section 6.6). While uncertainty remains, it has been recognized that many sources and components of PM 2.5 contribute to health effects. Overall, the results displayed in Table 6-18 indicate that many constituents of PM 2.5 can be linked with multiple health effects, and the evidence is not yet sufficient to allow differentiation of those constituents or sources that are more closely related to specific health outcomes.
Variability in the associations observed across PM 2.5 epidemiologic studies may be due in part to exposure error related to the use of county-level air quality data. Because western U.S. counties tend to be much larger and more topographically diverse than eastern U.S. counties, the day-to-day variations in concentration at one site, or even for the average of several sites, may not correlate well with the day-to-day variations in all parts of the county. For example, site-to-site correlations as a function of distance between sites (Section 3.5.1.2) fall off rapidly with distance in Los Angeles, but high correlations extend to larger distances in eastern cities such as Boston and Pittsburgh. These differences may be attributed to a number of factors including topography, the built environment, climate, source characteristics, ventilation usage, and personal activity patterns. For instance, regional differences in climate and infrastructure can affect time spent outdoors or indoors, air conditioning usage, and personal activity patterns. Characteristics of housing stock may also cause regional differences in effect estimates because new homes tend to have lower infiltration factors than older homes. Biases and uncertainties in exposure estimates resulting from these aspects can, in turn, cause bias and uncertainty in associated health effects estimates.
The new evidence reviewed in this ISA greatly expands upon the evidence available in the 2004 PM AQCD particularly in providing greater understanding of the underlying mechanisms for PM 2.5 induced cardiovascular and respiratory effects for both short- and long-term exposures. Recent studies have provided new evidence linking long-term exposure to PM 2.5 with cardiovascular outcomes that has expanded upon the continuum of effects ranging from the more subtle subclinical measures to cardiopulmonary mortality.
2.3.3. Exposure to PM10-2.5
2.3.3.1. Effects of Short-Term Exposure to PM 10-2.5

Cardiovascular Effects
Generally positive associations were reported between short-term exposure to PM 10-2.5 and hospital admissions or ED visits for cardiovascular causes. These results are supported by a large U.S. multicity study of older adults that reported PM 10-2.5 associations with CVD hospital admissions, and only a slight reduction in the PM 10-2.5 risk estimate when included in a copollutant model with PM 2.5 (Section 6.2.10). The PM 10-2.5 associations with cardiovascular hospital admissions and ED visits were observed in study locations with mean 24-h avg PM 10-2.5 concentrations ranging from 7.4 to 13 μg/m3. These results are supported by the associations observed between PM 10-2.5 and cardiovascular mortality in areas with 24-h avg PM 10-2.5 concentrations ranging from 6.1-16.4 μg/m3 (Section 6.2.11). The results of the epidemiologic studies were further confirmed by studies that examined dust storm events, which contain high concentrations of crustal material, and found an increase in cardiovascular-related ED visits and hospital admissions. Additional epidemiologic studies have reported PM 10-2.5 associations with other cardiovascular health effects including supraventricular ectopy and changes in HRV (Section 6.2.1.1). Although limited in number, studies of controlled human exposures provide some evidence to support the alterations in HRV observed in the epidemiologic studies (Section 6.2.1.2). The few toxicological studies that examined the effect of PM 10-2.5 on cardiovascular health effects used IT instillation due to the technical challenges in exposing rodents via inhalation to PM 10-2.5 , and, as a result, provide only limited evidence on the biological plausibility of PM 10-2.5 induced cardiovascular effects. The potential for PM 10-2.5 to elicit an effect is supported by dosimetry studies, which show that a large proportion of inhaled particles in the 3-6 micron (d ae ) range can reach and deposit in the lower respiratory tract, particularly the tracheobronchial (TB) airways (Figures 4-3 and 4-4). Collectively, the evidence from epidemiologic studies, along with the more limited evidence from controlled human exposure and toxicological studies is suggestive of a causal relationship between short-term exposures to PM 10-2.5 and cardiovascular effects.
Respiratory Effects
A number of recent epidemiologic studies conducted in Canada and France found consistent, positive associations between respiratory ED visits and hospital admissions and short-term exposure to PM 10-2.5 in studies with mean 24-h avg concentrations ranging from 5.6-16.2 μg/m3 (Section 6.3.8) . In these studies, the strongest relationships were observed among children, with less consistent evidence for adults and older adults (i.e., ≥ 65). In a large multicity study of older adults, PM 10-2.5 was positively associated with respiratory hospital admissions in both single and copollutant models with PM 2.5 . In addition, a U.S.-based multicity study found evidence for an increase in respiratory mortality upon short-term exposure to PM 10-2.5 , but these associations have not been consistently observed in single-city studies (Section 6.3.9). A limited number of epidemiologic studies have focused on specific respiratory morbidity outcomes, and found no evidence of an association with lower respiratory symptoms, wheeze, and medication use (Section 6.3.1.1). While controlled human exposure studies have not observed an effect on lung function or respiratory symptoms in healthy or asthmatic adults in response to short-term exposure to PM 10-2.5 , healthy volunteers have exhibited an increase in markers of pulmonary inflammation. Toxicological studies using inhalation exposures are still lacking, but pulmonary injury has been observed in animals after IT instillation exposure (Section 6.3.5.3). In some cases, PM 10-2.5 was found to be more potent than PM 2.5 and effects were not attributable to endotoxin. Both rural and urban PM 10-2.5 have induced inflammation and injury responses in rats or mice exposed via IT instillation, making it difficult to distinguish the health effects of PM 10-2.5 from different environments. Overall, epidemiologic studies, along with the limited number of controlled human exposure and toxicological studies that examined PM 10-2.5 respiratory effects provide evidence that is suggestive of a causal relationship between short- term exposures to PM 10-2.5 and respiratory effects.
Mortality
The majority of studies evaluated in this review provide some evidence for mortality associations with PM 10-2.5 in areas with mean 24-h avg concentrations ranging from 6.1-16.4 μg/m3. However, uncertainty surrounds the PM 10-2.5 associations reported in the studies evaluated due to the different methods used to estimate PM 10-2.5 concentrations across studies (e.g., direct measurement of PM 10-2.5 using dichotomous samplers, calculating the difference between PM 10 and PM 2.5 concentrations). In addition, only a limited number of PM 10-2.5 studies have investigated potential confounding by gaseous copollutants or the influence of model specification on PM 10-2.5 risk estimates.
A new U.S.-based multicity study, which estimated PM 10-2.5 concentrations by calculating the difference between the county-average PM 10 and PM 2.5 , found associations between PM 10-2.5 and mortality across the U.S., including evidence for regional variability in PM 10-2.5 risk estimates (Section 6.5.2.3). Additionally, the U.S.-based multicity study provides preliminary evidence for greater effects occurring during the warmer months (i.e., spring and summer). A multicity Canadian study provides additional evidence for an association between short-term exposure to PM 10-2.5 and mortality (Section 6.5.2.3). Although consistent positive associations have been observed across both multi- and single-city studies, more data are needed to adequately characterize the chemical and biological components that may modify the potential toxicity of PM 10-2.5 and compare the different methods used to estimate exposure. Overall, the evidence evaluated is suggestive of a causal relationship between short-term exposures to PM 10-2.5 and mortality.
2.3.4. Integration of PM 10-2.5 Effects
Epidemiologic, controlled human exposure, and toxicological studies have provided evidence that is suggestive for relationships between short-term exposure to PM 10-2.5 and cardiovascular effects, respiratory effects, and mortality. Conclusions regarding causation for the various health effects and outcomes were made for PM 10-2.5 as a whole regardless of origin, since PM 10-2.5 -related effects have been demonstrated for a number of different environments (e.g., cities reflecting a wide range of environmental conditions). Associations between short-term exposure to PM 10-2.5 and cardiovascular and respiratory effects, and mortality have been observed in locations with mean PM 10-2.5 concentrations ranging from 5.6 to 33.2 μg/m3, and maximum PM 10-2.5 concentrations ranging from 24.6 to 418.0 μg/m3) (Figure 2-3). A number of different health effects are included in Figure 2-3 to provide an integration of the range of effects by mean concentration, with a focus on cardiovascular and respiratory effects, and mortality (i.e., health effects categories with at least a suggestive causal determination). To date, a sufficient amount of evidence does not exist in order to draw conclusions regarding the health effects and outcomes associated with long-term exposure to PM 10-2.5 .
In epidemiologic studies, associations between short-term exposure to PM 10-2.5 and cardiovascular outcomes (i.e., IHD hospital admissions, supraventricular ectopy, and changes in HRV) have been found that are similar in magnitude to those observed in PM 2.5 studies. Controlled human exposure studies have also observed alterations in HRV, providing consistency and coherence for the effects observed in the epidemiologic studies. To date, only a limited number of toxicological studies have been conducted to examine the effects of PM 10-2.5 on cardiovascular effects. All of these studies involved IT instillation due to the technical challenges of using PM 10-2.5 for rodent inhalation studies. As a result, the toxicological studies evaluated provide limited biological plausibility for the PM 10-2.5 effects observed in the epidemiologic and controlled human exposure studies.
Figure 2-3. Summary of U.S. studies examining the association between short-term exposure to PM 10-2.5 and cardiovascular morbidity/mortality and respiratory morbidity/mortality. All effect estimates have been standardized to reflect a10 μg/m3 increase in mean 24-h avg PM 10-2.5 concentration and ordered by increasing concentration.

Limited evidence is available from epidemiologic studies for respiratory health effects and outcomes in response to short-term exposure to PM 10-2.5 . An increase in respiratory hospital admissions and ED visits has been observed, but primarily in studies conducted in Canada and Europe. In addition, associations are not reported for lower respiratory symptoms, wheeze, or medication use. Controlled human exposure studies have not observed an effect on lung function or respiratory symptoms in healthy or asthmatic adults, but healthy volunteers have exhibited pulmonary inflammation. The toxicological studies (all IT instillation) provide evidence of pulmonary injury and inflammation. In some cases, PM 10-2.5 was found to be more potent than PM 2.5 and effects were not solely attributable to endotoxin.
Currently, a national network is not in place to monitor PM 10-2.5 concentrations. As a result, uncertainties surround the concentration at which the observed associations occur. Ambient concentrations of PM 10-2.5 are generally determined by the subtraction of PM 10 and PM 2.5 measurements, using various methods. For example, some epidemiologic studies estimate PM 10-2.5 by taking the difference between collocated PM 10 and PM 2.5 monitors while other studies have taken the difference between county average PM 10 and PM 2.5 concentrations. Moreover, there are potential differences among operational flow rates and temperatures for PM 10 and PM 2.5 monitors used to calculate PM 10-2.5 . Therefore, there is greater error in ambient exposure to PM 10-2.5 compared to PM 2.5 . This would tend to increase uncertainty and make it more difficult to detect effects of PM 10-2.5 in epidemiologic studies. In addition, the various differences between eastern and western U.S. counties can lead to exposure misclassification, and the potential underestimation of effects in western counties (as discussed for PM 2.5 in Section 2.3.2).
It is also important to note that the chemical composition of PM 10-2.5 can vary considerably by location, but city-specific speciated PM 10-2.5 data are limited. PM 10-2.5 may contain Fe, Si, Al, and base cations from soil, plant and insect fragments, pollen, fungal spores, bacteria, and viruses, as well as fly ash, brake lining particles, debris, and automobile tire fragments.
The 2004 PM AQCD presented the limited amount of evidence available that examined the potential association between exposure to PM 10-2.5 and health effects and outcomes. The current evidence, primarily from epidemiologic studies, builds upon the results from the 2004 PM AQCD and indicates that short-term exposure to PM 10-2.5 is associated with effects on both the cardiovascular and respiratory systems. However, variability in the chemical and biological composition of PM 10-2.5 , limited evidence regarding effects of the various components of PM 10-2.5 , and lack of clearly defined biological mechanisms for PM 10-2.5 -related effects are important sources of uncertainty.
2.3.5. Exposure to UFPs
2.3.5.1. Effects of Short-Term Exposure to UFPs

Cardiovascular Effects
Controlled human exposure studies provide the majority of the evidence for cardiovascular health effects in response to short-term exposure to UFPs. While there are a limited number of studies that have examined the association between UFPs and cardiovascular morbidity, there is a larger body of evidence from studies that exposed subjects to fresh DE, which is typically dominated by UFPs. These studies have consistently demonstrated changes in vasomotor function following exposure to atmospheres containing relatively high concentrations of particles (Section 6.2.4.2). Markers of systemic oxidative stress have also been observed to increase after exposure to various particle types that are predominantly in the UFP size range. In addition, alterations in HRV parameters have been observed in response to controlled human exposure to UF CAPs, with inconsistent evidence for changes in markers of blood coagulation following exposure to UF CAPs and DE (Sections 6.2.1.2 and 6.2.8.2). A few toxicological studies have also found consistent changes in vasomotor function, which provides coherence with the effects demonstrated in the controlled human exposure studies (Section 6.2.4.3). Additional UFP-induced effects observed in toxicological studies include alterations in HRV, with less consistent effects observed for systemic inflammation and blood coagulation. Only a few epidemiologic studies have examined the effect of UFPs on cardiovascular morbidity and collectively they found inconsistent evidence for an association between UFPs and CVD hospital admissions, but some positive associations for subclinical cardiovascular measures (i.e., arrhythmias and supraventricular beats) (Section 6.2.2.1). These studies were conducted in the U.S. and Europe in areas with mean particle number concentration ranging from ~8,500 to 36,000 particles/cm3. However, UFP number concentrations are highly variable (i.e., concentrations drop off quickly from the road compared to accumulation mode particles), and therefore, more subject to exposure error than accumulation mode particles. In conclusion, the evidence from the studies evaluated is suggestive of a causal relationship between short-term exposures to UFPs and cardiovascular effects.
Respiratory Effects
A limited number of epidemiologic studies have examined the potential association between short-term exposure to UFPs and respiratory morbidity. Of the studies evaluated, there is limited, and inconsistent evidence for an association between short-term exposure to UFPs and respiratory symptoms, as well as asthma hospital admissions in locations a median particle number concentration of ~6,200 to a mean of 38,000 particles/cm3 (Section 6.3.10). The spatial and temporal variability of UFPs also affects these associations. Toxicological studies have reported respiratory effects including oxidative, inflammatory, and allergic responses using a number of different UFP types (Section 6.3). Although controlled human exposure studies have not extensively examined the effect of UFPs on respiratory outcomes, a few studies have observed small UFP-induced asymptomatic decreases in pulmonary function. Markers of pulmonary inflammation have been observed to increase in healthy adults following controlled exposures to UFPs, particularly in studies using fresh DE. However, it is important to note that for both controlled human exposure and animal toxicological studies of exposures to fresh DE, the relative contributions of gaseous copollutants to the respiratory effects observed remain unresolved. Thus, the current collective evidence is suggestive of a causal relationship between short-term exposures to UFPs and respiratory effects.
2.3.6. Integration of UFP Effects
The controlled human exposure studies evaluated have consistently demonstrated effects on vasomotor function and systemic oxidative stress with additional evidence for alterations in HRV parameters in response to exposure to UF CAPs. The toxicological studies provide coherence for the changes in vasomotor function observed in the controlled human exposure studies. Epidemiologic studies are limited because a national network is not in place to measure UFP in the U.S. UFP concentrations are spatially and temporally variable, which would increase uncertainty and make it difficult to detect associations between health effects and UFPs in epidemiologic studies. In addition, data on the composition of UFPs, the spatial and temporal evolution of UFP size distribution and chemical composition, and potential effects of UFP constituents are sparse.
More limited evidence is available regarding the effect of UFPs on respiratory effects. Controlled human exposure studies have not extensively examined the effect of UFPs on respiratory measurements, but a few studies have observed small decrements in pulmonary function and increases in pulmonary inflammation. Additional effects including oxidative, inflammatory, and pro- allergic outcomes have been demonstrated in toxicological studies. Epidemiologic studies have found limited and inconsistent evidence for associations between UFPs and respiratory effects.
Overall, a limited number of studies have examined the association between exposure to UFPs and morbidity and mortality. Of the studies evaluated, controlled human exposure and toxicological studies provide the most evidence for UFP-induced cardiovascular and respiratory effects; however, many studies focus on exposure to DE. As a result, it is unclear if the effects observed are due to UFP, larger particles (i.e., PM 2.5 ), or the gaseous components of DE. Additionally, UF CAPs systems are limited as the atmospheric UFP composition is modified when concentrated, which adds uncertainty to the health effects observed in controlled human exposure studies (Section 1.5.3).
2.4. Policy Relevant Considerations
2.4.1. Potentially Susceptible Populations
Upon evaluating the association between short- and long-term exposure to PM and various health outcomes, studies also attempted to identify populations that are more susceptible to PM (i.e., populations that have a greater likelihood of experiencing health effects related to exposure to an air pollutant (e.g., PM) due to a variety of factors including, but not limited to: genetic or developmental factors, race, gender, life stage, lifestyle (e.g., smoking status and nutrition) or preexisting disease; as well as, population-level factors that can increase an individual's exposure to an air pollutant (e.g., PM) such as socioeconomic status [SES], which encompasses reduced access to health care, low educational attainment, residential location, and other factors). These studies did so by conducting stratified analyses; by examining effects in individuals with an underlying health condition; or by developing animal models that mimic the pathophysiologic conditions associated with an adverse health effect. In addition, numerous studies that focus on only one potentially susceptible population provide supporting evidence on whether a population is susceptible to PM exposure. These studies identified a multitude of factors that could potentially contribute to whether an individual is susceptible to PM (Table 8-2). Although studies have primarily used exposures to PM 2.5 or PM 10 , the available evidence suggests that the identified factors may also enhance susceptibility to PM 10-2.5 . The examination of susceptible populations to PM exposure allows for the NAAQS to provide an adequate margin of safety for both the general population and for susceptible populations.
During specific periods of life (i.e., childhood and advanced age), individuals may be more susceptible to environmental exposures, which in turn can render them more susceptible to PM- related health effects. An evaluation of age-related health effects suggests that older adults have heightened responses for cardiovascular morbidity with PM exposure. In addition, epidemiologic and toxicological studies provide evidence that indicates children are at an increased risk of PM- related respiratory effects. It should be noted that the health effects observed in children could be initiated by exposures to PM that occurred during key windows of development, such as in utero. Epidemiologic studies that focus on exposures during development have reported inconsistent findings (Section 7.4), but a recent toxicological study suggests that inflammatory responses in pregnant women due to exposure to PM could result in health effects in the developing fetus.
Epidemiologic studies have also examined whether additional factors, such as gender, race, or ethnicity modify the association between PM and morbidity and mortality outcomes. Although gender and race do not seem to modify PM risk estimates, limited evidence from two studies conducted in California suggest that Hispanic ethnicity may modify the association between PM and mortality.
Recent epidemiologic and toxicological studies provided evidence that individuals with null alleles or polymorphisms in genes that mediate the antioxidant response to oxidative stress (i.e., GSTM1), regulate enzyme activity (i.e., MTHFR and cSHMT), or regulate levels of procoagulants (i.e., fibrinogen) are more susceptible to PM exposure. However, some studies have shown that polymorphisms in genes (e.g., HFE) can have a protective effect against effects of PM exposure. Additionally, preliminary evidence suggests that PM exposure can impart epigenetic effects (i.e., DNA methylation); however, this requires further investigation.
Collectively, the evidence from epidemiologic and toxicological, and to a lesser extent, controlled human exposure studies, indicate increased susceptibility of individuals with underlying CVDs and respiratory illnesses (i.e., asthma) to PM exposure. Controlled human exposure and toxicological studies provide additional evidence for increased PM-related cardiovascular effects in individuals with underlying respiratory health conditions.
Recently studies have begun to examine the influence of preexisting chronic inflammatory conditions, such as diabetes and obesity, on PM-related health effects. These studies have found some evidence for increased associations for cardiovascular outcomes along with pathophysiologic alterations in markers of inflammation, oxidative stress, and acute phase response. However, more research is needed to thoroughly examine the affect of PM exposure on obese individuals and to identify the biological pathway(s) that could increase the susceptibility of diabetic and obese individuals to PM.
There is also evidence that SES, measured using surrogates such as educational attainment or residential location, modifies the association between PM and morbidity and mortality outcomes. In addition, nutritional status, another surrogate measure of SES, has been shown to have protective effects against PM exposure in individuals that have a higher intake of some vitamins and nutrients.
Overall, the epidemiologic, controlled human exposure, and toxicological studies evaluated in this review provide evidence for increased susceptibility for various populations, including children and older adults, people with pre-existing cardiopulmonary diseases, and people with lower SES.
2.4.2. Lag Structure of PM-Morbidity and PM-Mortality Associations
Epidemiologic studies have evaluated the time-frame in which exposure to PM can impart a health effect. PM exposure-response relationships can potentially be influenced by a multitude of factors, such as the underlying susceptibility of an individual (e.g., age, pre-existing diseases), which could increase or decrease the lag times observed.
An attempt has been made to identify whether certain lag periods are more strongly associated with specific health outcomes. The epidemiologic evidence evaluated in the 2004 PM AQCD supported the use of lags of 0-1 days for cardiovascular effects and longer moving averages or distributed lags for respiratory diseases (U.S. EPA, 2004, 056905). However, currently, little consensus exists as to the most appropriate a priori lag times to use when examining morbidity and mortality outcomes. As a result, many investigators have chosen to examine the lag structure of associations between PM concentration and health outcome instead of focusing on a priori lag times. This approach is informative because if effects are cumulative, higher overall risks may exist than would be observed for any given single-day lag.
2.4.2.1. PM-Cardiovascular Morbidity Associations
Most of the studies evaluated that examined the association between cardiovascular hospital admissions and ED visits report associations with short-term PM exposure at lags 0- to 2-days, with more limited evidence for shorter durations (i.e., hours) between exposure and response for some health effects (e.g., onset of MI) (Section 6.2.10). However, these studies have rarely examined alternative lag structures. Controlled human exposure and toxicological studies provide biological plausibility for the health effects observed in the epidemiologic studies at immediate or concurrent day lags. Although the majority of the evidence supports shorter lag times for cardiovascular health effects, a recent study has provided preliminary evidence suggesting that longer lag times (i.e., 14- day distributed lag model) may be plausible for non-ischemic cardiovascular conditions (Section 6.2.10). Panel studies of short-term exposure to PM and cardiovascular endpoints have also examined the time frame from exposure to health effect using a wide range of lag times. Studies of ECG changes indicating ischemia show effects at lags from several hours to 2 days, while lag times ranging from hours to several week moving averages have been observed in studies of arrhythmias, vasomotor function and blood markers of inflammation, coagulation and oxidative stress (Section 6.2). The longer lags observed in these panel studies may be explained if the effects of PM are cumulative. Although few studies of cumulative effects have been conducted, toxicological studies have demonstrated PM-dependent progression of atherosclerosis. It should be noted that PM exposure could also lead to an acute event (e.g., infarction or stroke) in individuals with atherosclerosis that may have progressed in response to cumulative PM exposure. Therefore, effects have been observed at a range of lag periods from a few hours to several days with no clear evidence for any lag period having stronger associations then another.
2.4.2.2. PM-Respiratory Morbidity Associations
Generally, recent studies of respiratory hospital admissions that evaluate multiple lags, have found effect sizes to be larger when using longer moving averages or distributed lag models. For example, when examining hospital admissions for all respiratory diseases among older adults, the strongest associations were observed when using PM concentrations 2 days prior to the hospital admission (Section 6.3.8). Longer lag periods were also found to be most strongly associated with asthma hospital admissions and ED visits in children (3-5 days) with some evidence for more immediate effects in older adults (lags of 0 and 1 day), but these observations were not consistent across studies (Section 6.3.8). These variable results could be due to the biological complexity of asthma, which inhibits the identification of a specific lag period. The longer lag times identified in the epidemiologic studies evaluated are biologically plausible considering that PM effects on allergic sensitization and lung immune defenses have been observed in controlled human exposure and toxicological studies. These effects could lead to respiratory illnesses over a longer time course (e.g., within several days respiratory infection may become evident, resulting in respiratory symptoms or a hospital admission). However, inflammatory responses, which contribute to some forms of asthma, may result in symptoms requiring medical care within a shorter time frame (e.g., 0-1 days).
2.4.2.3. PM-Mortality Associations
Epidemiologic studies that focused on the association between short-term PM exposure and mortality (i.e., all-cause, cardiovascular, and respiratory) mostly examined a priori lag structures of either 1 or 0-1 days. Although mortality studies do not often examine alternative lag structures, the selection of the aforementioned a priori lag days has been confirmed in additional studies, with the strongest PM-mortality associations consistently being observed at lag 1 and 0-1-days (Section 6.5). However, of note is recent evidence for larger effect estimates when using a distributed lag model.
Epidemiologic studies that examined the association between long-term exposure to PM and mortality have also attempted to identify the latency period from PM exposure to death (Section 7.6.4). Results of the lag comparisons from several cohort studies indicate that the effects of changes in exposure on mortality are seen within five years, with the strongest evidence for effects observed within the first two years. Additionally, there is evidence, albeit from one study, that the mortality effect had larger cumulative effects spread over the follow-up year and three preceding years.
2.4.3. PM Concentration-Response Relationship
An important consideration in characterizing the PM-morbidity and mortality association is whether the concentration-response relationship is linear across the full concentration range that is encountered or if there are concentration ranges where there are departures from linearity (i.e., nonlinearity). In this ISA studies have been identified that attempt to characterize the shape of the concentration-response curve along with possible PM “thresholds” (i.e., levels which PM concentrations must exceed in order to elicit a health response). The epidemiologic studies evaluated that examined the shape of the concentration-response curve and the potential presence of a threshold have focused on cardiovascular hospital admissions and ED visits and mortality associated with short-term exposure to PM 10 and mortality associated with long-term exposure to PM 2.5 .
A limited number of studies have been identified that examined the shape of the PM- cardiovascular hospital admission and ED visit concentration-response relationship. Of these studies, some conducted an exploratory analysis during model selection to determine if a linear curve most adequately represented the concentration-response relationship; whereas, only one study conducted an extensive analysis to examine the shape of the concentration-response curve at different concentrations (Section 6.2.10.10). Overall, the limited evidence from the studies evaluated supports the use of a no-threshold, log-linear model, which is consistent with the observations made in studies that examined the PM-mortality relationship.
Although multiple studies have previously examined the PM-mortality concentration-response relationship and whether a threshold exists, more complex statistical analyses continue to be developed to analyze this association. Using a variety of methods and models, most of the studies evaluated support the use of a no-threshold, log-linear model; however, one study did observe heterogeneity in the shape of the concentration-response curve across cities (Section 6.5). Overall, the studies evaluated further support the use of a no-threshold log-linear model, but additional issues such as the influence of heterogeneity in estimates between cities, and the effect of seasonal and regional differences in PM on the concentration-response relationship still require further investigation.
In addition to examining the concentration-response relationship between short-term exposure to PM and mortality, Schwartz et al. (2008, 156963) conducted an analysis of the shape of the concentration-response relationship associated with long-term exposure to PM. Using a variety of statistical methods, the concentration-response curve was found to be indistinguishable from linear, and, therefore, little evidence was observed to suggest that a threshold exists in the association between long-term exposure to PM 2.5 and the risk of death (Section 7.6)
2.4.4. PM Sources and Constituents Linked to Health Effects
Recent epidemiologic, toxicological, and controlled human exposure studies have evaluated the health effects associated with ambient PM constituents and sources, using a variety of quantitative methods applied to a broad set of PM constituents, rather than selecting a few constituents a priori (Section 6.6). There is some evidence for trends and patterns that link particular ambient PM constituents or sources with specific health outcomes, but there is insufficient evidence to determine whether these patterns are consistent or robust.
For cardiovascular effects, multiple outcomes have been linked to a PM 2.5 crustal/soil/road dust source, including cardiovascular mortality and ST-segment changes. Additional studies have reported associations between other sources (i.e., traffic and wood smoke/vegetative burning) and cardiovascular outcomes (i.e., mortality and ED visits). Studies that only examined the effects of individual PM 2.5 constituents found evidence for an association between EC and cardiovascular hospital admissions and cardiovascular mortality. Many studies have also observed associations between other sources (i.e., salt, secondary SO 4 2–/long-range transport, other metals) and cardiovascular effects, but at this time, there does not appear to be a consistent trend or pattern of effects for those factors.
There is less consistent evidence for associations between PM sources and respiratory health effects, which may be partially due to the fact that fewer source apportionment studies have been conducted that examined respiratory-related outcomes (e.g., hospital admissions) and measures (e.g., lung function). However, there is some evidence for associations between respiratory ED visits and decrements in lung function with secondary SO 4 2– PM 2.5 . In addition, crustal/soil/road dust and traffic sources of PM have been found to be associated with increased respiratory symptoms in asthmatic children and decreased PEF in asthmatic adults. Inconsistent results were observed in those PM 2.5 studies that used individual constituents to examine associations with respiratory morbidity and mortality, although Cu, Pb, OC, and Zn were related to respiratory health effects in two or more studies.
A few studies have identified PM 2.5 sources associated with total mortality. These studies found an association between mortality and the PM 2.5 sources: secondary SO 4 2–/long-range transport, traffic, and salt. In addition, studies have evaluated whether the variation in associations between PM 2.5 and mortality or PM 10 and mortality reflects differences in PM 2.5 constituents. PM 10 - mortality effect estimates were greater in areas with a higher proportion of Ni in PM 2.5 , but the overall PM 10 -mortality association was diminished when New York City was excluded in sensitivity analyses in two of the studies. V was also found to modify PM 10 -mortality effect estimates. When examining the effect of species-to-PM 2.5 mass proportion on PM 2.5 -mortality effect estimates, Ni, but not V, was also found to modify the association.
Overall, the results indicate that many constituents of PM can be linked with differing health effects and the evidence is not yet sufficient to allow differentiation of those constituents or sources that are more closely related to specific health outcomes. These findings are consistent with the conclusions of the 2004 PM AQCD (U.S. EPA, 2004, 056905) (i.e., that a number of source types, including motor vehicle emissions, coal combustion, oil burning, and vegetative burning, are associated with health effects). Although the crustal factor of fine particles was not associated with mortality in the 2004 PM AQCD (U.S. EPA, 2004, 056905), recent studies have suggested that PM (both PM 2.5 and PM 10-2.5 ) from crustal, soil or road dust sources or PM tracers linked to these sources are associated with cardiovascular effects. In addition, PM 2.5 secondary SO 4 2– has been associated with both cardiovascular and respiratory effects.
2.5. Welfare Effects
This section presents key conclusions and scientific judgments regarding causality for welfare effects of PM as discussed in Chapter 9. The effects of particulate NO X and SO X have recently been evaluated in the ISA for Oxides of Nitrogen and Sulfur – Ecological Criteria (U.S. EPA, 2008, 157074). That ISA focused on the effects from deposition of gas- and particle-phase pollutants related to ambient NO X and SO X concentrations that can lead to acidification and nutrient enrichment. Thus, emphasis in Chapter 9 is placed on the effects of airborne PM, including NO X and SO X , on visibility and climate, and on the effects of deposition of PM constituents other than NO X and SO X , primarily metals and carbonaceous compounds. EPA’s framework for causality, described in Chapter 1, was applied and the causal determinations are highlighted.

2.5.1. Summary of Effects on Visibility
Visibility impairment is caused by light scattering and absorption by suspended particles and gases. There is strong and consistent evidence that PM is the overwhelming source of visibility impairment in both urban and remote areas. EC and some crustal minerals are the only commonly occurring airborne particle components that absorb light. All particles scatter light, and generally light scattering by particles is the largest of the four light extinction components (i.e., absorption and scattering by gases and particles). Although a larger particle scatters more light than a similarly shaped smaller particle of the same composition, the light scattered per unit of mass is greatest for particles with diameters from ~0.3-1.0 μm.
For studies where detailed data on particle composition by size are available, accurate calculations of light extinction can be made. However, routinely available PM speciation data can be used to make reasonable estimates of light extinction using relatively simple algorithms that multiply the concentrations of each of the major PM species by its dry extinction efficiency and by a water growth term that accounts for particle size change as a function of relative humidity for hygroscopic species (e.g., sulfate, nitrate, and sea salt). This permits the visibility impairment associated with each of the major PM components to be separately approximated from PM speciation monitoring data.
Direct optical measurement of light extinction measured by transmissometer, or by combining the PM light scattering measured by integrating nephelometers with the PM light absorption measured by an aethalometer, offer a number of advantages compared to algorithm estimates of light extinction based on PM composition and relative humidity data. The direct measurements are not subject to the uncertainties associated with assumed scattering and absorption efficiencies used in the PM algorithm approach. The direct measurements have higher time resolution (i.e., minutes to hours), which is more commensurate with visibility effects compared with calculated light extinction using routinely available PM speciation data (i.e., 24-h duration).
Particulate sulfate and nitrate have comparable light extinction efficiencies (haze impacts per unit mass concentration) at any relative humidity value. Their light scattering per unit mass concentration increases with increasing relative humidity, and at sufficiently high humidity values (RH>85%) they are the most efficient particulate species contributing to haze. Particulate sulfate is the dominant source of regional haze in the eastern U.S. (>50% of the particulate light extinction) and an important contributor to haze elsewhere in the country (>20% of particulate light extinction). Particulate nitrate is a minor component of remote-area regional haze in the non-California western and eastern U.S., but an important contributor in much of California and in the upper Midwestern U.S., especially during winter when it is the dominant contributor to particulate light extinction.
EC and OC have the highest dry extinction efficiencies of the major PM species and are responsible for a large fraction of the haze, especially in the northwestern U.S., though absolute concentrations are as high in the eastern U.S. Smoke plume impacts from large wildfires dominate many of the worst haze periods in the western U.S. Carbonaceous PM is generally the largest component of urban excess PM 2.5 (i.e., the difference between urban and regional background concentration). Western urban areas have more than twice the average concentrations of carbonaceous PM than remote areas sites in the same region. In eastern urban areas PM 2.5 is dominated by about equal concentrations of carbonaceous and sulfate components, though the usually high relative humidity in the East causes the hydrated sulfate particles to be responsible for about twice as much of the urban haze as that caused by the carbonaceous PM.
PM 2.5 crustal material (referred to as fine soil) and PM 10-2.5 are significant contributors to haze for remote areas sites in the arid southwestern U.S. where they contribute a quarter to a third of the haze, with PM 10-2.5 usually contributing twice that of fine soil. Coarse mass concentrations are as high in the Central Great Plains as in the deserts though there are no corresponding high concentrations of fine soil as in the Southwest. Also the relative contribution to haze by the high coarse mass in the Great Plains is much smaller because of the generally higher haze values caused by the high concentrations of sulfate and nitrate PM in that region.
Visibility has direct significance to people’s enjoyment of daily activities and their overall sense of wellbeing. For example, psychological research has demonstrated that people are emotionally affected by poor VAQ such that their overall sense of wellbeing is diminished. Urban visibility has been examined in two types of studies directly relevant to the NAAQS review process: urban visibility preference studies and urban visibility valuation studies. Both types of studies are designed to evaluate individuals’ desire for good VAQ where they live, using different metrics. Urban visibility preference studies examine individuals’ preferences by investigating the amount of visibility degradation considered unacceptable, while economic studies examine the value an individual places on improving VAQ by eliciting how much the individual would be willing to pay for different amounts of VAQ improvement.
There are three urban visibility preference studies and two additional pilot studies that have been conducted to date that provide useful information on individuals’ preferences for good VAQ in the urban setting. The completed studies were conducted in Denver, Colorado, two cities in British Columbia, Canada, and Phoenix, AZ. The additional studies were conducted in Washington, DC. The range of median preference values for an acceptable amount of visibility degradation from the 4 urban areas was approximately 19-33 dv. Measured in terms of visual range (VR), these median acceptable values were between approximately 59 and 20 km.
The economic importance of urban visibility has been examined by a number of studies designed to quantify the benefits (or willingness to pay) associated with potential improvements in urban visibility. Urban visibility valuation research was described in the 2004 PM AQCD (U.S. EPA, 2004, 056905) and the 2005 PM Staff Paper (U.S. EPA, 2005, 090209). Since the mid-1990s, little new information has become available regarding urban visibility valuation (Section 9.2.4).
Collectively, the evidence is sufficient to conclude that a causal relationship exists between PM and visibility impairment.
2.5.2. Summary of Effects on Climate
Aerosols affect climate through direct and indirect effects. The direct effect is primarily realized as planet brightening when seen from space because most aerosols scatter most of the visible spectrum light that reaches them. The Intergovernmental Panel on Climate Change (IPCC) Fourth Assessment Report (AR4) (IPCC, 2007, 092765), hereafter IPCC AR4, reported that the radiative forcing from this direct effect was -0.5 (±0.4) W/m2 and identified the level of scientific understanding of this effect as 'Medium-low'. The global mean direct radiative forcing effect from individual components of aerosols was estimated for the first time in the IPCC AR4 where they were reported to be (all in W/m2 units): -0.4 (±0.2) for sulfate, -0.05 (±0.05) for fossil fuel-derived organic carbon, +0.2 (±0.15) for fossil fuel-derived black carbon (BC), +0.03 (±0.12) for biomass burning, -0.1 (±0.1) for nitrates, and -0.1 (±0.2) for mineral dust. Global loadings of anthropogenic dust and nitrates remain very troublesome to estimate, making the radiative forcing estimates for these constituents particularly uncertain.
Numerical modeling of aerosol effects on climate has sustained remarkable progress since the time of the 2004 PM AQCD (U.S. EPA, 2004, 056905), PM AQCD, though model solutions still display large heterogeneity in their estimates of the direct radiative forcing effect from anthropogenic aerosols. The clear-sky direct radiative forcing over ocean due to anthropogenic aerosols is estimated from satellite instruments to be on the order of -1.1 (±0.37) W/m2 while model estimates are -0.6 W/m2. The models' low bias over ocean is carried through for the global average: global average direct radiative forcing from anthropogenic aerosols is estimated from measurements to range from -0.9 to -1.9 W/m2, larger than the estimate of -0.8 W/m2 from the models.
Aerosol indirect effects on climate are primarily realized as an increase in cloud brightness (termed the 'first indirect' or Twomey effect), changes in precipitation, and possible changes in cloud lifetime. The IPCC AR4 reported that the radiative forcing from the Twomey effect was -0.7 (range: -1.1 to +4) and identified the level of scientific understanding of this effect as “Low” in part owing to the very large unknowns concerning aerosol size distributions and important interactions with clouds. Other indirect effects from aerosols are not considered to be radiative forcing.
Taken together, direct and indirect effects from aerosols increase Earth's shortwave albedo or reflectance thereby reducing the radiative flux reaching the surface from the Sun. This produces net climate cooling from aerosols. The current scientific consensus reported by IPCC AR4 is that the direct and indirect radiative forcing from anthropogenic aerosols computed at the top of the atmosphere, on a global average, is about -1.3 (range: -2.2 to -0.5) W/m2. While the overall global average effect of aerosols at the top of the atmosphere and at the surface is negative, absorption and scattering by aerosols within the atmospheric column warms the atmosphere between the Earth's surface and top of the atmosphere. In part, this is owing to differences in the distribution of aerosol type and size within the vertical atmospheric column since aerosol type and size distributions strongly affect the aerosol scattering and reradiation efficiencies at different altitudes and atmospheric temperatures. And, although the magnitude of the overall negative radiative forcing at the top of the atmosphere appears large in comparison to the analogous IPCC AR4 estimate of positive radiative forcing from anthropogenic GHG of about +2.9 (± 0.3) W/m 2, the horizontal, vertical, and temporal distributions and the physical lifetimes of these two very different radiative forcing agents are not similar; therefore, the effects do not simply off-set one another.
Overall, the evidence is sufficient to conclude that a causal relationship exists between PM and effects on climate, including both direct effects on radiative forcing and indirect effects that involve cloud feedbacks that influence precipitation formation and cloud lifetimes.
2.5.3. Summary of Ecological Effects of PM
Ecological effects of PM include direct effects to metabolic processes of plant foliage; contribution to total metal loading resulting in alteration of soil biogeochemistry and microbiology, plant growth and animal growth and reproduction; and contribution to total organics loading resulting in bioaccumulation and biomagnification across trophic levels. These effects were well- characterized in the 2004 PM AQCD (U.S. EPA, 2004, 056905). Thus, the summary below builds upon the conclusions provided in that review.
PM deposition comprises a heterogeneous mixture of particles differing in origin, size, and chemical composition. Exposure to a given concentration of PM may, depending on the mix of deposited particles, lead to a variety of phytotoxic responses and ecosystem effects. Moreover, many of the ecological effects of PM are due to the chemical constituents (e.g., metals, organics, and ions) and their contribution to total loading within an ecosystem.
Investigations of the direct effects of PM deposition on foliage have suggested little or no effects on foliar processes, unless deposition levels were higher than is typically found in the ambient environment. However, consistent and coherent evidence of direct effects of PM has been found in heavily polluted areas adjacent to industrial point sources such as limestone quarries, cement kilns, and metal smelters (Sections 9.4.3 and 9.4.5.7). Where toxic responses have been documented, they generally have been associated with the acidity, trace metal content, surfactant properties, or salinity of the deposited materials.
An important characteristic of fine particles is their ability to affect the flux of solar radiation passing through the atmosphere, which can be considered in both its direct and diffuse components. Foliar interception by canopy elements occurs for both up- and down-welling radiation. Therefore, the effect of atmospheric PM on atmospheric turbidity influences canopy processes both by radiation attenuation and by changing the efficiency of radiation interception in the canopy through conversion of direct to diffuse radiation. Crop yields can be sensitive to the amount of radiation received, and crop losses have been attributed to increased regional haze in some areas of the world such as China (Section 9.4.4). On the other hand, diffuse radiation is more uniformly distributed throughout the canopy and may increase canopy photosynthetic productivity by distributing radiation to lower leaves. The enrichment in photosynthetically active radiation (PAR) present in diffuse radiation may offset a portion of the effect of an increased atmospheric albedo due to atmospheric particles. Further research is needed to determine the effects of PM alteration of radiative flux on the growth of vegetation in the U.S.
The deposition of PM onto vegetation and soil, depending on its chemical composition, can produce responses within an ecosystem. The ecosystem response to pollutant deposition is a direct function of the level of sensitivity of the ecosystem and its ability to ameliorate resulting change. Many of the most important ecosystem effects of PM deposition occur in the soil. Upon entering the soil environment, PM pollutants can alter ecological processes of energy flow and nutrient cycling, inhibit nutrient uptake, change ecosystem structure, and affect ecosystem biodiversity. The soil environment is one of the most dynamic sites of biological interaction in nature. It is inhabited by microbial communities of bacteria, fungi, and actinomycetes, in addition to plant roots and soil macro-fauna. These organisms are essential participants in the nutrient cycles that make elements available for plant uptake. Changes in the soil environment can be important in determining plant and ultimately ecosystem response to PM inputs.
There is strong and consistent evidence from field and laboratory experiments that metal components of PM alter numerous aspects of ecosystem structure and function. Changes in the soil chemistry, microbial communities and nutrient cycling, can result from the deposition of trace metals. Exposures to trace metals are highly variable, depending on whether deposition is by wet or dry processes. Although metals can cause phytotoxicity at high concentrations, few heavy metals (e.g., Cu, Ni, Zn) have been documented to cause direct phytotoxicity under field conditions. Exposure to coarse particles and elements such as Fe and Mg are more likely to occur via dry deposition, while fine particles, which are more often deposited by wet deposition, are more likely to contain elements such as Ca, Cr, Pb, Ni, and V. Ecosystems immediately downwind of major emissions sources can receive locally heavy deposition inputs. Phytochelatins produced by plants as a response to sublethal concentrations of heavy metals are indicators of metal stress to plants. Increased concentrations of phytochelatins across regions and at greater elevation have been associated with increased amounts of forest injury in the northeastern U.S.
Overall, the ecological evidence is sufficient to conclude that a causal relationship is likely to exist between deposition of PM and a variety of effects on individual organisms and ecosystems, based on information from the previous review and limited new findings in this review. However, in many cases, it is difficult to characterize the nature and magnitude of effects and to quantify relationships between ambient concentrations of PM and ecosystem response due to significant data gaps and uncertainties as well as considerable variability that exists in the components of PM and their various ecological effects.
2.5.4. Summary of Effects on Materials
Building materials (metals, stones, cements, and paints) undergo natural weathering processes from exposure to environmental elements (wind, moisture, temperature fluctuations, sunlight, etc.). Metals form a protective film of oxidized metal (e.g., rust) that slows environmentally induced corrosion. However, the natural process of metal corrosion is enhanced by exposure to anthropogenic pollutants. For example, formation of hygroscopic salts increases the duration of surface wetness and enhances corrosion.
A significant detrimental effect of particle pollution is the soiling of painted surfaces and other building materials. Soiling changes the reflectance of opaque materials and reduces the transmission of light through transparent materials. Soiling is a degradation process that requires remediation by cleaning or washing, and, depending on the soiled surface, repainting. Particulate deposition can result in increased cleaning frequency of the exposed surface and may reduce the usefulness of the soiled material.
Attempts have been made to quantify the pollutant exposure levels at which materials damage and soiling have been perceived. However, to date, insufficient data are available to advance the knowledge regarding perception thresholds with respect to pollutant concentration, particle size, and chemical composition. Nevertheless, the evidence is sufficient to conclude that a causal relationship exists between PM and effects on materials.
2.6. Summary of Health Effects and Welfare Effects
Causal Determinations
This chapter has provided an overview of the underlying evidence used in making the causal determinations for the health and welfare effects and PM size fractions evaluated. This review builds upon the main conclusions of the last PM AQCD (U.S. EPA, 2004, 056905):
- "A growing body of evidence both from epidemiological and toxicological studies supports the general conclusion that PM 2.5 (or one or more PM 2.5 components), acting alone and/or in
- “A much more limited body of evidence is suggestive of associations between short-term (but not long-term) exposures to ambient coarse-fraction thoracic particles and various mortality and morbidity effects observed at times in some locations. This suggests that PM 10-2.5 , or some constituent component(s) of PM 10-2.5 , may contribute under some circumstances to increased human health risks with somewhat stronger evidence for associations with morbidity (especially respiratory) endpoints than for mortality.” (pg 9-79 and 9-80)
- "Impairment of visibility in rural and urban areas is directly related to ambient concentrations of fine particles, as modulated by particle composition, size, and hygroscopic characteristics, and by relative humidity.” (pg 9-99)
- “Available evidence, ranging from satellite to in situ measurements of aerosol effects on incoming solar radiation and cloud properties, is strongly indicative of an important role in climate for aerosols, but this role is still poorly quantified.” (pg 9-111)
The evaluation of the epidemiologic, toxicological, and controlled human exposure studies published since the completion of the 2004 PM AQCD have provided additional evidence for PM-related health effects. Table 2-6 provides an overview of the causal determinations for all PM size fractions and health effects. Causal determinations for PM and welfare effects, including visibility, climate, ecological effects, and materials are included in Table 2-7. Detailed discussions of the scientific evidence and rationale for these causal determinations are provided in the subsequent chapters of this ISA.

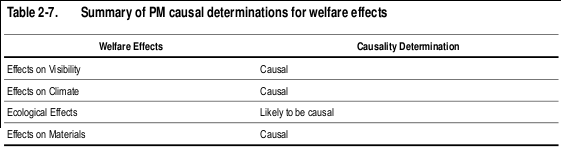
|
it could be completely unrelated
but I have had serious respiratory distress for 10 days.
everyone keeps saying it must be allergies.... but I do over look our lovely considerate pollution valley.
Yesterday (Sunday) the Air Products factory was making the worst loudest nasty noise I'd ever heard from them (in 10 years of listening to their crap). of course it wAS a Sunday....
If you read the report you know Cleveland is killing you.
If you read the report you know Cleveland is killing you.
I noticed the monitor data has been way down since Sunday (although at least one downtown monitor seems to always be out of service) - the weather has much to do with pollution being washed and blown from your window so the last two days should have been better. Also, when I drove by Mittal today it seems like they have cut back on the polluting - keep an eye on their stacks...
I don;t know of any specific dangerous emissions from Air Products but I am checking into all the pollution inventory reports and all the monitors... even the PM 10 monitors they say we don't need any more... we'll soon know more about the air we are breathing.
Disrupt IT
well...
we're moving, so god bless the next poor souls who more to the glorious Tremont....
Smart - we are too... from University Circle
Smart - we are too... from University Circle... as we save 1,000,000s of other lives.
Power to realNEO!
Disrupt IT
And I blame Frank...
And I blame Frank...
How long has he been in charge of this disgrace????
Disrupt IT
Action Jackson?
he's not in charge.... he couldn't run a quicky mart...
He is in charge of our local environmental policy, and it sucks
He is in charge of our local environmental policy, and it sucks. Trust me.
Disrupt IT